Dr Arun Srivastava – The AAV Club: Applying AAV Vectors to Gene Therapy
The sci-fi vision of gene therapy for curing genetic diseases is fast becoming a reality as more therapies are entering clinical and commercial development. Dr Arun Srivastava and his team at the University of Florida are investigating the use of the next generation of AAV vectors for gene therapy.
The Scope and Limitations of Gene Therapy
The notion of ‘gene therapy’ has intrigued scientists, biotechnologists, clinicians, futurists and the public for decades. The ‘biopunk’ subgenre of science fiction has long exploited society’s fascination with this paradigm, and the prospect of medical enhancement by means of genetic engineering has provided a rich theme in both utopian and dystopian visions of the future. However, gene therapy is no longer science fiction, but is fast becoming ‘science fact’.
Many diseases are driven by defective genes, leading to expression of dysfunctional proteins. Gene therapy aims to deliver fully-functioning genes to specific tissues, replacing or counteracting malfunctioning genes, thus driving sufficient expression of the functional protein. Gene therapy has its roots in the work of pioneering molecular biologist, Joshua Lederberg, who discovered that genetic material is transferred between bacteria by ‘conjugation’ – a primitive version of bacterial sex. Later, Lederberg with Norton Zinder discovered another mechanism of bacterial genetic transfer. They found that bacteriophages – small viruses that infect bacteria –also transfer genetic material between bacteria, known as ‘transduction’. In transduction, DNA or RNA become incorporated in the bacterial genome.
The first proof-of-concept model for human gene therapy was developed by husband-and-wife team, Waclaw and Elizabeth Szybalski, who demonstrated viral transduction in cultured human bone marrow cell lines. This showed the unthinkable – that viral transduction is also possible in eukaryotic cells (complex cells with a nucleus and membrane-bound compartments)! Transduction into the host cell’s nuclear genome, using viruses as delivery vectors, became the preferred method for gene therapy. While the concept is simple, the practicalities of delivering functional genes to targeted tissues, while evading immune responses, and maintaining a long-term effect, are challenging.
Currently, over 1800 approved clinical trials involving gene therapy have been conducted or are underway globally.
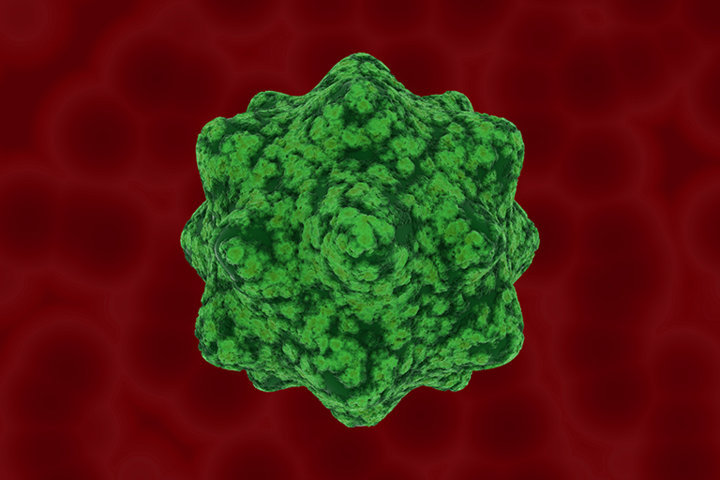
‘My work is focused on a harmless virus that causes no known disease. This virus, called AAV, is being used as a vehicle to deliver healthy genes into the body to treat, and hopefully cure, a wide variety of human diseases.’
Investigating AAV and B19 Parvoviruses for Gene Delivery
For almost four decades, Dr Srivastava and his team at the University of Florida College of Medicine have investigated the biology of human parvoviruses as potential vectors for gene therapy. Parvoviruses are a group of some of the smallest viruses that infect animals, with a virion (virus particle) diameter of only 25 nanometres – tiny even by viral standards! Dr Srivastava and his lab have focused their research efforts on two human parvoviruses – the adeno-associated virus (AAV) and the parvovirus B19 (also called primate erythroparvovirus 1). Both AAV and B19 are prevalent in the human population and relatively harmless, with AAV regarded as non-pathogenic, and the mildly-pathogenic B19 being responsible for common childhood rash. The small size, genomic simplicity and relative non-pathogenicity of the human parvoviruses make them ideal vectors for human gene therapy. Dr Srivastava’s group is interested in the application of human parvovirus transduction of blood cells for the gene therapy of haematological diseases, and they are working hard to make this a reality! Their major focus is treatment of haemoglobin deficiencies with AAV6 vectors. They are also focussing on the application of AAV3 vectors for treating haemophilia (clotting factor disorders), and hepatocellular carcinoma and hepatoblastoma (liver cancers) as an alternative to chemotherapy.
Dr Srivastava’s interest in parvoviruses began early, and rather serendipitously. As a first-year graduate student, he ‘became hooked’ when he stumbled upon a 1974 Nature paper on the mechanism of single-stranded DNA (ssDNA) replication, by the famous prokaryote taxonomist, Dr Thomas Cavalier-Smith. ‘Later, I learned that a non-pathogenic human parvovirus, the adeno-associated virus (AAV) contains ssDNA,’ he explains. Without these formative inspirations, Dr Srivastava may never have pioneered the application of parvoviruses as vectors for gene therapy. ‘I started working on AAV in 1980, and have continued ever since.’
The wild-type adeno-associated virus 2 (AAV2 serotype) was discovered in 1965. About 90% of humans have anti-AAV2 antibodies, implying that most of us have been exposed to AAV2, yet there is no conclusive evidence that infection leads to any known disease. Pioneering studies undertaken by Dr Srivastava in the 1980s elucidated the remarkable simplicity of the AAV genome and proteome. The AAV genome is a small linear ssDNA molecule containing 4680 nucleotides (for the AAV2 serotype), which encodes four replication proteins, three capsid proteins, and an assembly activating protein.
For development of viral gene therapy vectors capable of effectively entering cells, discovery of viral-specific receptor protein molecules on the surface membrane of target cells is important. Receptors are like ‘doorways’ into cells, allowing viruses to enter. In 1996, Dr Srivastava and colleagues demonstrated that AAV infection of cells is receptor-mediated, having identified a cell line (human megakaryocytic leukaemia cells, MB-02) resistant to AAV infection as it lacks specific receptors. This finding sparked a quest for the discovery of cell surface receptors indicative of transduction success, which became a major endeavour for the team. In 1999, they identified a cell receptor associated with AAV2 infection – human fibroblast growth factor receptor 1 (FGFR1) as a cellular co-receptor for AAV. In 2003, they discovered a co-receptor – a5b1 integrin – as a cellular co-receptor for parvovirus B19, and in 2010, identified human hepatocyte growth factor receptor (HGFR) as a cellular co-receptor for AAV3. As well as AAV2, the group is also investigating transduction efficiencies and tissue-specificities of other virus serotypes. While AAV2 is ideal for liver- and retina-directed gene therapy, Dr Srivastava and other groups have found that AAV3 and AAV6 are more effective for human liver and blood cells, respectively.
Blood clotting (coagulation) after an injury prevents bleeding and promotes wound healing – vital to prevent blood loss and haemorrhaging. Therefore, conditions affecting clotting are particularly dangerous. Haemophilia is a debilitating inherited disease that impairs the blood’s ability to clot, and sufferers are susceptible to prolonged post-injury bleeding, easy bruising and increased risk of internal bleeding in the joints and brain – potentially causing severe, permanent damage. Blood clotting is a complex pathway involving several specialised blood proteins. These include blood clotting factor proteins produced in liver hepatocyte cells that facilitate the clotting process. There are two types of haemophilia – haemophilia A and haemophilia B – that result in the deficiencies or absence of clotting factors VIII and IX, respectively. A number of clinical trials are currently underway investigating the clinical efficacy of clotting factor replacement by gene therapy. These involve the simple procedure of infusion of the therapeutic AAV viruses into the hepatic artery of patients (delivers blood to the liver) for delivery of corrective genes that encode deficient clotting factors to the hepatocytes. Dr Srivastava’s lab focusses on clotting factor IX replacement for haemophilia B treatment in hepatocytes, using ‘humanised’ mouse and non-human primate models.
Engineering AAV Vectors for Gene Therapy
To overcome the limitations of the wild-type AAV, recombinant strategies, involving genetic modification of the virus, have been deployed by Dr Srivastava’s group to improve the delivery, transduction and expression of corrective genes to target tissues. Ironically, while high dosages of AAV virus are typically necessary for efficient gene therapy, high dosages can also activate T-cell mediated immune responses that attack the viral vector. Therefore, a major component of Dr Srivastava’s work is to improve transduction efficiency, so that curative outcomes can be achieved at lower viral dosages.
A major aim of the lab is to elucidate cellular mechanisms that attack and destroy the viral vector. Inside cells, unwanted proteins destined for destruction are tagged with ubiquitin – a regulatory protein that serves as a signal for protease enzymes to break down unwanted proteins. Dr Srivastava’s group discovered that this is responsible for around 80% of AAV2 vectors failing to enter the nucleus, and are trying to circumventing this destructive pathway to improve gene therapy efficiency.
The group found that the presence of tyrosine, serine or threonine residues (amino acids prone to phosphorylation) on the AAV capsid surface destines the virus to ubiquitination-mediated destruction. Hence, they have genetically modified wild-type AAV viruses, by mutating these surface amino acids to phenylalanine or valine (both amino acids), which do not undergo phosphorylation, and thus bypass the cell’s ubiquitination-based defences. This strategy proved highly effective for improving transduction rates. Tyrosine-to-phenylalanine mutation in AAV2 led to 10-times lower viral doses needed for adequate transduction in mice. While mouse-based models are standard in Dr Srivastava’s lab, the group are shifting the focus of their modified AAV studies from mouse models to human models, as the next step to feasible human gene therapy. This poses a challenge, as AAV transduction efficiencies have typically been much lower in human cells than in mouse cells. The AAV3 serotype, once rejected as a potential vector because of poor in vivo transduction in mouse livers, has turned out to be effective in transducing human hepatocytes. The group has therefore focussed on genetically engineering the AAV3 virus to further increase transduction rates in human hepatocytes. In 2016, the group demonstrated that just two mutations, of a serine and threonine to valine, in AAV3 gave 8 times and 80 times greater transduction rates, respectively, than AAV8 and AAV5 (two favoured gene therapy vectors for clinical development) in human hepatocytes in a ‘humanised’ mouse model.
Even if transduction is successful, and the virally-delivered gene-of-interest has been delivered to the target cell, there is no guarantee that the corresponding corrective protein will be sufficiently produced (‘expressed’) to cure the target disease. While the ssDNA is a defining feature of AAV as a naturally occurring virus, Dr Srivastava, along with others in the AAV community, speculate that engineering the wild-type single-stranded AAV (ssAAV) to have a double-stranded ‘self-complimentary’ genome can improve corrective gene expression through improved transcription (transfer of genetic information from DNA to RNA).
Single-stranded DNA is transcriptionally-inactive, and the viral synthesis of a second strand is a rate-limiting step in cell culture studies. Unfortunately, second strand DNA synthesis is typically suppressed by host cells, often leading to only 5% of mouse hepatocytes expressing the protein after transduction. Dr Srivastava’s group has identified a cellular chaperone protein (involved in folding newly-expressed proteins), called FKBP52, phosphorylated forms of which inhibit the second-strand synthesis by binding to AAV ssDNA. Indeed, the vast majority of AAV gene therapy clinical trials have been carried out using ssAAV. Several research groups, including Dr Srivastava’s, are working on developing next-generation AAV gene therapy vectors.
In Dr Srivastava’s group, hepatocytes are not the only targets for AAV gene therapy for clotting disorders. The group is also interested in AAV transduction of hematopoietic stem/progenitor cells (HSPCs) as agents of gene and cell therapy. HSPCs are stem cells produced in bone marrow that differentiate to form all other types of blood cells (multipotency). They therefore have greater therapeutic scope, with potential to treat beta-globin deficiencies, leukodystrophies, immunodeficiencies and even AIDS. HSPCs are a promising choice for autologous stem cell/gene therapy. This would involve extracting HSPCs from patients, in vitro culturing the extracted HSPCs, and viral transduction (gene delivery, gene editing) to introduce the corrective gene into the cultured HSPCs. Next, the modified HSPCs are inserted back into the same patient so they differentiate into the functional cells of interest. Alternatively, the modified HSPCs may be differentiated in culture by adding certain chemicals before infusion into patients. For the development of feasible stem cell/gene therapies, efficient transduction procedures must be established. Unfortunately, poor transduction and corrective protein expression in HSPCs are hampering their applicability to cell therapy. In 2016, the group demonstrated a novel highly efficient approach to viral transduction of HSPCs using AAV6 vectors. These insights are highly promising, and could be the basis of feasible high-efficiency transduction and gene editing approaches.
AAV Gene Therapy – the Way Forward
Dr Srivastava’s group has pioneered the application of AAV gene therapy in pre-clinical models, identifying AAV-specific cellular co-receptors and in vivo mechanisms that may hamper gene delivery, and engineering wild-type vectors for improved robustness. The group hopes to continue further optimisation of the AAV genome and capsid proteins, and improve the tissue-specificity of different vectors for more targeted therapy. There is overwhelming evidence for the safety and efficacy of recombinant AAV gene therapy with 162 clinical trials conducted to date.
Meet the researcher
Professor Arun Srivastava
Cancer & Genetics Research Complex
Departments of Pediatrics
University of Florida
Gainesville
USA
Professor Arun Srivastava is Chief of the Division of Cellular & Molecular Therapy in the Departments of Pediatrics, and the Powell Gene Therapy Center, at the University of Florida. He received his PhD from the Indian Institute of Science in Bangalore, India. After completing his postdoctoral training at the Memorial Sloan-Kettering Cancer Center in New York, he worked as a Research Associate at the University of Florida, before a two-decade sojourn at Indiana University School of Medicine, Indianapolis, where he rose to the rank of Professor. He then returned to the University of Florida in 2004. He has served on various NIH Study Sections, and is also on the editorial boards of several esteemed gene therapy-related journals. He was appointed as Honorary Professor and Advisor, Shenzhen Institute of Xiangya Biomedicine, Central South University, Shenzhen, PR China, and University of Florida Research Foundation Professor, and Children’s Miracle Network Scholar. For nearly four decades, Dr Srivastava’s research has focused on the virology and pathophysiology of two human parvoviruses, the adeno-associated virus (AAV), and the parvovirus B19, and the application of these as vectors for gene therapy. His laboratory has shed light on many fascinating insights into the field of parvoviruses, including molecular mechanisms of viral entry into cells, intracellular viral trafficking and regulation of AAV DNA replication and encapsidation, and parvovirus-induced pathogenicity in transgenic and knockout mouse models.
CONTACT
E: aruns@peds.ufl.edu
T: (+1) 352 273 8259
W: http://research.pediatrics.med.ufl.edu/researchers/research-faculty/arun-srivastava/
KEY COLLABORATORS
Dr Chen Ling, University of Florida
Dr Mavis Agbandje-McKenna, University of Florida
Dr Roland Herzog, University of Florida
Dr Mervin Yoder, Indiana University
Dr Emmanuel Payen, University of Paris
Dr Philippe Leboulch, University of Paris
Dr Alok Srivastava, Christian Medical College
Dr Barry Byrne, University of Florida
FUNDING
NIH R01 HL-097088
NIH R21 AI-131328
NIH R41 AI-122735
Children’s Miracle Network
Kitzman Endowment