Dr Ashley Cowart – Small Scale Roots of Metabolic Disease
Although metabolic diseases plague Western countries, we still don’t fully understand the molecular mechanisms that trigger these diseases. Therefore, biochemists such as Dr Ashley Cowart at the Medical University of South Carolina strive to uncover the cellular pathways behind such diseases, in the hope of finding new treatments.
According to the World Health Organisation, a body mass index of over 25 means that a person is overweight, whereas one over 30 indicates obesity. Both of these categories are major risk factors in cardiovascular and metabolic diseases. Moreover, the International Diabetes Federation reports that in 2012, almost 400 million people worldwide were living with diabetes – a number that is expected to climb to more than half a billion people by 2030. A whopping 80% of these patients are located in developed countries, and the incidence of type 2 diabetes continues to rise in both Europe and the United States. This type of diabetes is strongly linked to abdominal obesity and can be confirmed based on hyperglycaemia in blood plasma. Although metabolic ailments such as obesity and diabetes manifest on a large scale, scientists have found that they start small – on a cellular level. In order to understand and treat these diseases, researchers such as Dr Ashley Cowart and her colleagues are exploring the interactions and behaviours of a group of molecules called ‘sphingolipids’ inside living cells.
Sphingolipids are a class of lipid that contain an 18-carbon amino-alcohol, called a ‘sphingoid base’. This basic structure has several variations, each giving rise to a unique member of the sphingolipid family such as sphingosine, sphingosine-1-phosphate, ceramide, sphingomyelin and glycosphingolipids. ‘This class of compounds was discovered by Johann Thudichum who described them in his book, A Treatise on the Chemical Constitution of the Brain, published in 1884,’ Dr Cowart tells us. ‘At that time, sphingolipids were thought of simply as insulation for the nervous system, similar to the rubber coating on electrical wiring. It was not until the late 1900s that scientists found that these lipids were dynamically generated or broken down in response to stress, and that they bound to protein targets in the cell to elicit cell stress responses.’ In Thudichum’s experiments, he noted that these lipids must somehow be chemically distinct from other lipids. Because at that time he did not understand the composition and structures of these odd lipids, he named them after the mythological Sphinx.
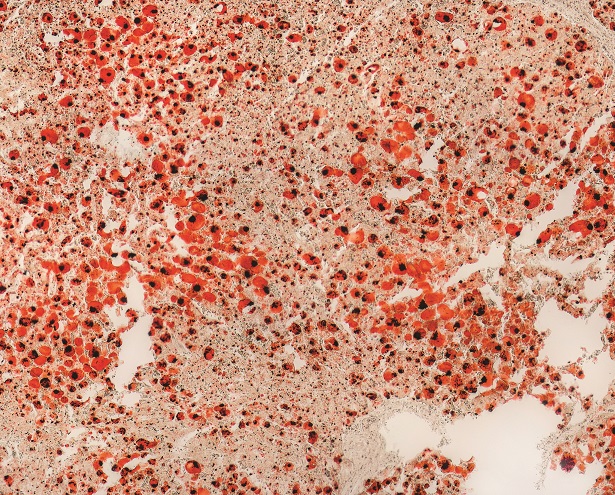
‘This class of compounds was discovered by Johann Thudichum who described them in his book published in 1884. At that time, sphingolipids were thought of simply as insulation for the nervous system, similar to the rubber coating on electrical wiring. It was not until the late 1900s that scientists found that these lipids were dynamically generated or broken down in response to stress, and that they bound to protein targets in the cell to elicit cell stress responses.’
Solving a Lipidic Riddle
Sphingolipids possess special signalling and structural properties, and these compounds have been found to play major roles in the behaviour of tissues affected by diabetes. In the past few decades, research has shown that sphingolipids are more than just simple structural components of cell membranes – they also help to control numerous crucial processes, such as growth, differentiation, chemical signalling and programmed cell death or apoptosis. In addition, these lipids trigger insulin resistance – an initial step in diabetes. They have also been found to promote inflammation associated with diabetes and are now thought to mediate outcomes of diabetes including non-alcoholic fatty liver disease and cardiovascular disease.
When sphingolipids become abnormally regulated, they can trigger cell death and even dysfunction of vital organs such as the heart and the pancreas, or in the aetiology of cancer and multiple sclerosis. Moreover, researchers have found clues suggesting that the levels of sphingolipids in blood plasma may act as a warning sign that the process of gaining insulin resistance has begun. However, while many new discoveries have emerged in the field of sphingolipid research in the past few decades, their precise roles remain obscure. Thus, there is an urgent need for the development of novel experimental approaches to uncover the exact molecular mechanisms that lead to changes in sphingolipid function, so that new treatments can be developed.
Motivated by the immense impact of these lipids on human health and life expectancy, Dr Cowart and her colleagues investigate the ways that dietary and plasma fats affect the metabolism of sphingolipids present in tissues. This is important because the concentration of lipids in plasma increases in obesity and diabetes, and this increase can change the sphingolipid content in many tissues, such as those in the heart, liver, adipose tissue and skeletal muscles. The team wondered if these lipids may contribute to tissue illness such as insulin resistance, inflammation, abnormal growth of cardiac muscle, and fatty liver disease.
To test their hypothesis, Dr Cowart and her colleagues created experimental lab setups using rodent and tissue culture models. Through the course of these experiments, the team found that rodents placed on high saturated fat diets had dramatic changes in specific sphingolipids in their tissues. These changes resulting from high-fat diets can trigger major problems in gene regulation and chemical signalling pathways. By creating a novel mouse model based on high saturated fat-feeding, Dr Cowart and colleagues have been able to pinpoint several facts.
‘Essentially, this line of work began with our findings published in 2009 that excess saturated fat does not drive sphingolipid biosynthesis in a non-specific way, as was the going thinking at the time, but that sphingolipid metabolic pathways reconfigure in response to saturated fats, which suggests that sphingolipid synthesis may in part mediate stress responses to dyslipidemia,’ Dr Cowart explains. ‘Our studies in the liver, muscle, and heart have mechanistically linked this “reconfiguration” to cell processes including inflammation, maladaptive autophagy, endoplasmic reticulum stress and apoptosis. Most of the earlier studies were in skeletal muscle or skeletal muscle model systems, then we moved into cardiac muscle, and we have some more recent work in liver.’
Research Directions in Lipidomics
In a series of papers published between 2008 and 2016 in some of the most reputable scientific journals, Dr Cowart’s team reported many new insights into the roles and normal and abnormal functions of sphingolipids in tissues. Some of the team’s findings were related to the behaviour of cardiac tissue in diabetes, the pathway of myocardial sphingolipids, the phenomenon of hepatic inflammation caused by diets rich in saturated fats and mediated by sphingolipids, and the role of saturated fats in the stimulation of genes responsible for promoting steatosis, or fatty liver disease.
Type 2 diabetes can trigger changes to the heart tissue of otherwise healthy individuals. Although they display none of the factors of traditional heart disease, these people can suffer from diabetic cardiomyopathy – an abnormal enlargement of the cardiac muscle that vastly increases the risk of heart failure. In mouse models of diabetic cardiomyopathy, heart dysfunction and enlargement happen due to a lipid overload caused by dietary saturated fatty acids. At the same time, this phenomenon occurs in the presence of the synthesis of new sphingolipids. However, it was not clear whether the effects are caused by saturated fatty acids and sphingolipids in general, or by certain species of lipid.
Dr Cowart’s team showed that diets rich in saturated fatty acids caused cardiac muscle enlargement, functional systolic and diastolic problems of the left ventricle, and intracellular degradation leading to cell death. Furthermore, they discovered a specific metabolic pathway involving dietary saturated fatty acids and sphingolipids, which together cause lipotoxicity and ultimately heart disease. More precisely, the fatty acid myristate was found to induce cell degradation and hypertrophy by its metabolism to sphingolipids, while palmitate did not. Finally, Dr Cowart’s group was able to determine that these effects occurred through specifically the (dihydro)ceramide synthase 5 form.
The sphingolipid base backbone formation is catalysed by an enzyme called serine palmitoyltransferase (SPT), which, if inhibited, reduces cardiac disease appearance in those with lipid overload. However, the metabolites involved in these phenomena were not previously known, along with their relevance for living tissue. Dr Cowart and her colleagues sought to determine whether the so-called d16 sphingolipids derived from myristate occurred in the myocardial tissue and if their metabolism was different from that of sphingolipids derived from palmitate. The study found that the bases of d16 lipids are present in more than a third of the sphingolipids in the myocardium. The team also found that dietary saturated fats promoted their production. Moreover, only d16 sphingolipids and not d18 contributed to cell death and chemical changes in tissue. This was the second specific sphingolipid pathway that Dr Cowart and colleagues identified, and they now propose that either of these pathways may serve in the future as therapeutic targets for diabetic heart pathology.
Fatty hepatitis – or steatohepatitis – is present in about 20% of patients suffering from fatty liver disease and results in cirrhosis, fibrosis, and risk of hepatocellular carcinoma. Having already demonstrated that lipid overload stimulates the production of sphingosine kinase 1 (SphK1), an enzyme responsible for generating sphingosine-1-phosphate (S1P), Dr Cowart and her team sought to test whether S1P promotes the inflammation of the liver in obesity. The team discovered a twofold increase of the enzyme in human livers affected by non-alcoholic fatty liver disease, a finding which was confirmed in mouse models with high-fat diets. All mice livers also showed inflammatory factors and infiltration of immune cells – all except for the mice without SphK1. Additionally, the team found that SphK1 is promoted in muscle tissue by palmitate, and that the levels of interleukin-6, an immune modulator, were significantly lower in obese mice lacking SphK1.
The team’s investigations have paved the way to a better understanding of the action of sphingosine kinase 1 in dietary lipid overload, and offer future therapeutic targets for treating individuals suffering from metabolic disease.
Meet the researcher
Dr L. Ashley Cowart
Department of Biochemistry and Molecular Biology
Medical University of South Carolina
Charleston, South Carolina
USA
Dr L. Ashley Cowart obtained her PhD in biochemistry in 2001 from the Vanderbilt University in Nashville, Tennessee. She is now an Associate Professor at the Department of Biochemistry and Molecular Biology at the Medical University of South Carolina and also a research health scientist at the Ralph H. Johnson VA Medical Centre. Her team studies how plasma lipids affect tissue sphingolipid metabolism, and their roles in mediating diabetes-associated disease. Dr Cowart, who also serves as the co-Director of the Lipidomics Core Facility at the Medical University of South Carolina, is a member of the American Society for Biochemistry and Molecular Biology, serves on multiple committees in both the lRalph H. Johnson VA Medical Center and the Medical University of South Carolina, and has published over 40 papers in peer-reviewed journals.
CONTACT
E: cowartl@musc.edu
T: (+1) 843 876 2787
W: http://academicdepartments.musc.edu/biochemistry/faculty/cowartl.htm
FUNDING
Dr Cowart’s laboratory is funded through both the NIH and the VA.
REFERENCES
T Geng, L Xia, S Russo, D Kamara, LA Cowart, Prosteatotic genes are associated with unsaturated fat suppression of saturated fat-induced hepatic steatosis in C57BL/6 mice, Nutrition Research, 2015, 35, 812–22.
T Geng, A Sutter, MD Harland, BA Law, JS Ross, D Lewin, A Palanisamy, SB Russo, KD Chavin, LA Cowart, SphK1 mediates hepatic inflammation in a mouse model of NASH induced by high saturated fat feeding and initiates proinflammatory signaling in hepatocytes, Journal of Lipid Research, 2015, 56, 2359–71.
JS Ross, W Hu, B Rosen, AJ Snider, LM Obeid, LA Cowart, Sphingosine kinase 1 is regulated by peroxisome proliferator-activated receptor α in response to free fatty acids and is essential for skeletal muscle interleukin-6 production and signaling in diet-induced obesity, Journal of Biological Chemistry, 2013, 288, 22193–206.
SB Russo, R Tidhar, AH Futerman, LA Cowart, Myristate-derived d16:0 sphingolipids constitute a cardiac sphingolipid pool with distinct synthetic routes and functional properties, Journal of Biological Chemistry, 2013, 288, 13397–409.