Dr Scott Hsu and Dr F. Douglas Witherspoon – Plasma guns fire into the race for fusion
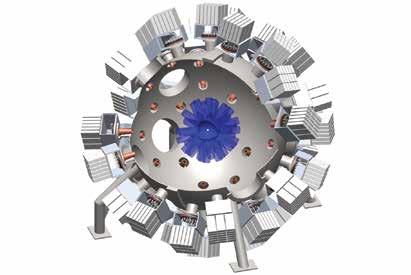
What drew you towards nuclear fusion research?
I remember reading about fusion in high school and thinking that fusion energy is something that the world will eventually need. This was in the mid-1980s when achieving a burning plasma in the laboratory felt imminent. At UCLA I had the fortune to take two plasmaphysics courses with Professor Francis Chen (author of the famous textbook) and work in his lab. He alerted me to a summer internship at the Princeton Plasma Physics Laboratory, where I solidified my desire to pursue a research career in fusion. I got into Princeton’s graduate program in plasma physics in 1993 and, as they say, the rest is history.
How did you come to work on magnetoinertial fusion?
I always had a keen interest in alternatefusion concepts. I went to Los Alamos to work on the solid-liner magnetized target fusion collaboration between LANL and the Air Force Research Laboratory. I quickly came to see the challenges of solid liners. The destructive nature of imploding a solid liner and its relatively slow implosion speed (demanding long-lived targets that are hard to create) contributed to a slow rate of research progress. Later I came to learn about Dr. Y. C. Francis Thio’s proposed plasma-liner approach to magneto-inertial fusion (MIF), which could potentially overcome many of MIF’s crippling problems. With Thio’s encouragement, I organized a national workshop at Los Alamos in 2008. This led to the formation of my present research team and a winning proposal to the DOE Office of Fusion Energy Sciences to construct the Plasma Liner Experiment (PLX) and to initiate a programme researching the feasibility of forming plasma liners by merging supersonic plasma jets.
What is the project you lead and who are the principal investigators?
I lead the ARPA-E-funded PLX-α project, comprised of LANL, HyperV Technologies Corp. (F. Douglas Witherspoon), the University of Alabama in Huntsville (Jason Cassibry), the University of New Mexico (Mark Gilmore), Tech-X Corporation (Peter Stoltz), and Brookhaven National Laboratory (Roman Samulyak). They have all been involved in plasma-liner and/or plasma-jet research since the inception of the plasma guns project in 2004 or the PLX project in 2010. The recently initiated three-year ARPA-E project will execute two experimental campaigns. The first nine-jet campaign covers an octant of our spherical chamber. The purpose is to show that a portion of a liner with sufficient quality can be formed via merging jets. If this is successful, we will conduct full spherical implosions with up to 60 jets. What we hope to learn are the scalings of uniformity and ram-pressure with convergence, the initial jet parameters, and the number of jets. These results will be used to evaluate and benchmark our computer codes, which can then be used to design full-scale plasma-jet-driven magneto-inertial fusion (PJMIF) experiments.
What promising results have you obtained?
Our prior experiments focused on characterizing the evolution of a single plasma jet propagating from the chamber wall to the merging radius and the oblique merging of two jets. From the single-jet experiments, we learned that jet propagation leads to tolerable expansion and density degradation. We measured jet parameters and profiles necessary for faithful simulations. From the two-jet-merging experiments, we learned that oblique shocks indeed form during the merging process and must be characterized in more detail in order for us to assess whether their effects are acceptable for PJMIF. Overall, our initial experiments and modeling efforts have established the scientific basis for plasma-liner formation via merging plasma jets, and encouraged us to proceed to an experimental demonstration of spherical plasma-liner formation.
How does PJMIF compare to other approaches?
One attribute of PJMIF is the low-cost MIF part of it, as compared to magnetic fusion energy (MFE) or inertial confinement fusion (ICF). We feel that lowering fusion R&D costs is essential to the timely development of fusion energy. Another is that PJMIF is a reactor-friendly embodiment of MIF that seeks to overcome the disadvantages of many other MIF approaches, such as their destructive nature and/or slow implosion velocity. The plasma guns that launch the jets will not be damaged, and their electrodes have a slow erosion rate. We can fire dozens of shots per day for faster R&D progress. In a reactor, PJMIF can in theory fire at 1 Hz, resulting in attractive power-plant economics. Another key advantage of PJMIF over MFE and ICF is the potential compatibility with flowing liquid blankets, which would serve as the hightemperature medium for breeding tritium and generating electricity, avoiding an open-ended, costly solid-materials development program.
How can PJMIF achieve that?
By using a strong magnetic field to slow down the rate of energy loss, ignition can be achieved at densities higher than MFE but lower than ICF. In this intermediate range, the combination of plasma energy and power density allows for optimum cost to generate ignited plasma (200 million USD compared to the billions to tens of billions required for mainstream fusion approaches). The plasma implodes along with a magnetic field strong enough to retard heat loss. These arguments were elegantly derived from physics first principles in a paper by retired LANL scientists Irv Lindemuth and Richard Siemon, “The fundamental parameter space of controlled thermonuclear fusion,” meant for a broad audience beyond plasma-physics experts. The non-destructive, standoff, and high-shotrate nature of PJMIF is enabled by the innovative plasma guns that launch the required plasma jets for PJMIF to reach ignition conditions.
Will PJMIF reach ignition sooner than competing technologies?
If things go well, then maybe yes! However, if there is one lesson to be learned from the history of fusion research, it is that there are always unforeseeable problems, which become harder close to ignition. PJMIF enables a lowcost, high-shot-rate, rapid-learning pathway toward ignition. If ignition is achieved, PJMIF has attractive features for scaling up to a fusion power reactor. These are the main reasons we are excited by and are motivated to develop PJMIF.
Developing a cheaper, faster pathway to economical fusion power
A multi-institutional research programme is underway to develop a novel approach to fusion power in the form of plasma-jet-driven magneto-inertial fusion (PJMIF).
PJMIF – A NOVEL APPROACH TO FUSION
The concept of PJMIF was invented by Thio in the 1990s. It involves the use of plasma jets fired at supersonic velocities by plasma guns, which are arranged in a spherical, inward-pointing array, aimed towards a target area. The guns fire simultaneously, launching a spherical array of plasma jets that merge to create a spherically imploding plasma liner composed of a heavy element such as argon. The plasma liner compresses a magnetised deuterium-tritium (DT) plasma target to the high temperatures and pressures required for fusion reactions. Other approaches to fusion are notably different from PJMIF in terms of reactor shapes and technologies, plasma conditions, and energy and cost requirements. ICF consists of a small, solid DT pellet that is compressed and superheated inside a cylindrical cavity by very high-power lasers positioned in a radial configuration. MFE uses torus-shaped magnetic fields to confine and heat a very large plasma, such as the tokamak. Developing a cheaper, faster pathway to economical fusion power A multi-institutional research programme is underway to develop a novel approach to fusion power in the form of plasma-jet-driven magneto-inertial fusion (PJMIF). For PJMIF, the magnetised DT plasma target is situated in the centre of a chamber, where it is compressed by the imploding liner. The liner is formed from low-temperature plasma jets travelling at supersonic speed, originating from the electromagnetic plasma guns mounted on the walls of a spherical vacuum chamber. In an orchestrated sequence of precisely timed events, the magnetised target is first created by firing only a subset of jets containing DT fuel, and in the split second that the plasma fuel comes together it is magnetised by external lasers. It is after this that the target is compressed by the trailing plasma liner. Alternatively PJMIF can be targetless, in which case the fuel is carried at the leading edge of the plasma liner itself and magnetised in a similar manner. Plasma-liner dynamics are currently being investigated in the new PLX-α experiment, to demonstrate the viability of this approach and investigate how to scale up to larger reactors on the path to ignition – the “holy grail” of fusion research. For PJMIF this occurs when fusion reactions alone generate much more energy than that required to form and accelerate the plasma jets. The energy for sustained fusion would be generated solely by the fusing atomic nuclei in the plasma. Hsu also works in nuclear weapons science at LANL, affording him valuable insights into fusion ignition that most other fusion-energy researchers do not have.
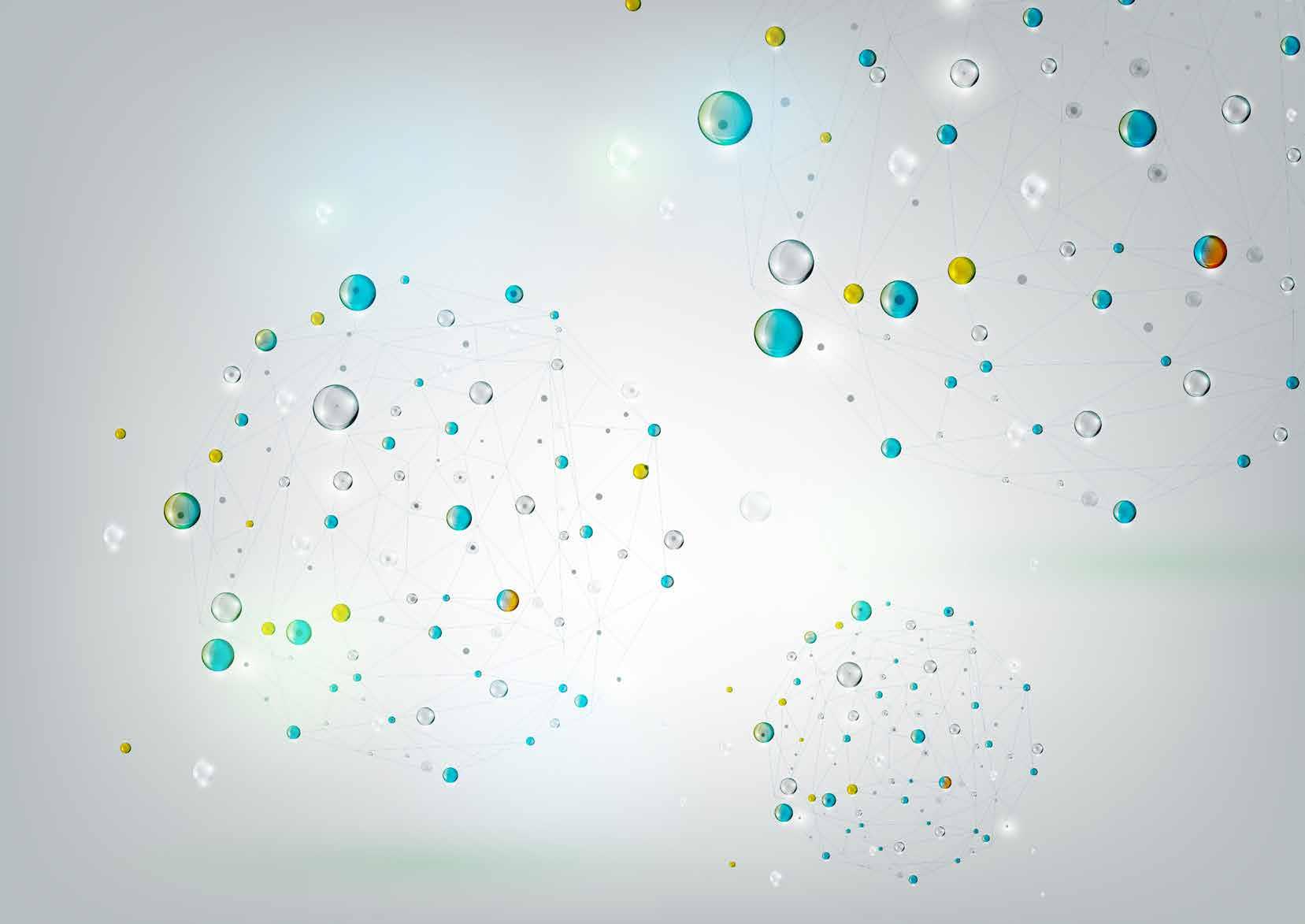
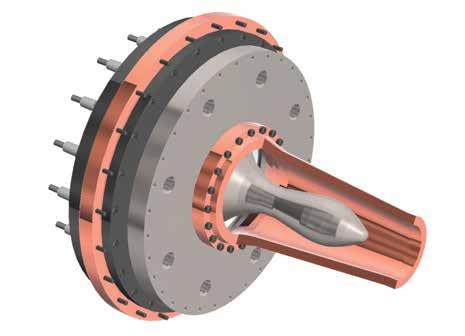
APPROACH ADVANTAGES
PJMIF aims to hit an important “sweet spot” with a plasma density in the range of 1018- 1023 cm ˉ3. As Hsu explains, “fusion ignition can be achieved in a pulsed system at densities much higher than those of MFE and much lower than those of ICF.” To achieve ignition in this density range, a strong magnetic field is required to slow down energy loss from the plasma target. With an ideal plasma density for ignition at 1021 cmˉ3, this sweet spot in “thermonuclear parameter space” affords an optimal driverfacility cost of a few hundred million U.S. dollars, in contrast to the tens of billions U.S. dollars required for MFE or ICF. PLX, started in 2010, involved extensive computer modeling, theoretical analysis, and single- and double-jet experiments. Single-jet experiments showed that the expansion and evolving density profile of the jets is within acceptable bounds. The double-jet experiments investigated the behaviour of jet merging. The new PLX-α experiment that is now underway will first look at the behaviour of nine plasma jets. When supersonic jets merge, they form shock waves that heat and change the shape and profile of the liner. This can disrupt the uniformity of the implosion and cause liner heating, potentially leading to reduced ability of the liner to compress a magnetised target. Jet merging effects will be scrutinised in the PLX-α experiment, to pave the way for the hundreds of jets that surround the origin in a spherical arrangement in the fully realised reactor. Larger numbers of jets are favourable because they provide better implosion uniformity and lower per-gun power requirements. In future PJMIF experiments the magnetic field in the target may give a greater degree of stability against the destabilising effects of liner nonuniformities, as compared to an approach like ICF. MIF approaches that use a solid liner can be highly destructive to experimental equipment generating the high-energy fusion processes. Destructive approaches require more maintenance and additional equipment costs, which slows research by reducing the experimental shot rate. One of the strong benefits of PJMIF is that it is non-destructive, and the electrodes used to accelerate the plasma jets have a very slow erosion rate, enabling a much higher shot rate.
FUTURE DIRECTIONS
The hydrodynamic and magneto-hydrodynamic (MHD) computational codes used to model the jet propagation and plasma-liner implosion, similar to those for describing the behaviour of stars, are not fully predictive at this point. Hsu and his team bravely tackle this challenge through carefully planned research: “The detailed nature of the plasma-liner formation and implosion processes are indeed difficult to predict via theory or computations alone, in part due to sensitivity to jet initial conditions, which are imperfectly known, and in part due to imperfect models in the codes. Our experimental R&D plan will systematically remove these uncertainties in a phased development plan”. For example, this is precisely the purpose of the first nine-jet phase of the experiment. Radiation effects are to be included in radiation-MHD models which “selfconsistently describe the leading-order physics of PJMIF”. The required coaxial plasma guns were also invented by Thio. They are designed and built by HyperV Technologies Corp., where experimental validation of gun performance and engineering advancements are led by Witherspoon. Today’s state-of-the-art plasma guns are still in the process of being scaled up to accelerate jets with larger masses and higher densities and to achieve better control over the mass and density profiles of the jets. In terms of scalability, a large array of guns will need to function in a reproducible and reliable manner. These new experiments will provide essential data for planning the road ahead and, in addition, inform the direction of simulations, which go hand in hand with experimental work. The best simulations of PJMIF so far are those reported by Charles Knapp and Ron Kirkpatrick. They use a one-dimensional model of the imploding plasma liner with radiation- and MHD equations. Dr. Hsu informs us that “these unoptimized 1D simulations are an indication of what is achievable, showing that energy gains of >30 may be possible.” The energy gain is a parameter that is paramount for this line of research. It is defined as the ratio between the energy output and the energy input. The bigger this ratio is, the more energy the reactor produces for a given cost. Hsu and his partners hope that success in their ongoing work will lead to private investment in furthering PJMIF development for economical fusion power.
Meet the Researchers
Dr Scott Hsu
Scientist, Physics Division Los Alamos National Laboratory
Dr F. Douglas Witherspoon
President & Chief Scientist of HyperV Technologies Corp.
Dr. Scott Hsu (Ph.D., Princeton University) leads the PLX-α project. He is an experimental plasma physicist with a background in magnetic fusion energy science, basic plasma physics, plasma astrophysics, and high-energy-density plasma physics. He has worked in these fields for 20+ years. His experience stretches from fundamental topics like magnetic reconnection to multiple areas of fusion research. In 2002, he was a winner of the American Physical Society’s Award for Excellence in Plasma Physics Research.
CONTACT
T: (505) 667-3386
Dr. Doug Witherspoon is an experimental plasma physicist with 30+ years of research experience in start-up companies dedicated to applying plasma technologies to industrial, defense, space, and energy applications. He has extensive experience in pulsed-power and plasma-driven accelerators, with seven patents awarded. He leads the gun development effort at HyperV Technologies Corp., a small company dedicated to the development of commercially viable fusion-energy technologies.
CONTACT
T: 703-378-4882
Key Collaborators
Professor Jason Cassibry, University of Alabama in Huntsville
Dr. Samuel Brockington, HyperV Technologies Corp.
Dr. Andrew Case, HyperV Technologies Corp.
Professor Mark Gilmore, University of New Mexico
Dr. Roman Samulyak, Brookhaven National Laboratory
Dr. Peter Stoltz, Tech-X Corporation FUNDING ARPA-E (U. S. Department of Energy)
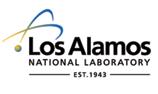
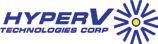
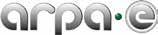
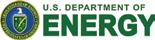