Professor Andrew Webb – Tumours In The Crosshairs: New Tools To Resurrect An Old Strategy For Targeted Cancer Therapy
Professor Andrew Webb and his undergraduate research students at Wellesley College have developed a highly selective nano-therapeutic that could be used to treat solid tumours. The method involves gold nanoparticles loaded with boron-10, which can selectively accumulate in tumours. Irradiation with a beam of neutrons causes these boron atoms to become unstable and decompose, releasing high-energy alpha particles and lithium atoms that destroy the tumour.
Treatment of solid tumours. At the cutting edge of nanotechnology
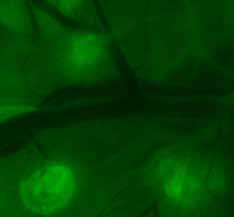
The bright green spots indicate multiple human tumour xenografts stained for blood vessels
The classical and most widely used approaches for treating cancer are surgery, radiotherapy and chemotherapy, and more recent approaches include immunotherapy and hormonal therapies. Surgery is not always possible, is highly invasive, and can sometimes lead to complications, such as post-operative infections. Conventional radiotherapy involves irradiating a tumour with beams of radiation to shrink it in size or cause its complete destruction. It is often used as a supplementary therapy, in conjunction with surgery and/or chemotherapy. However, current limitations include localised side-effects, caused by the destruction of healthy tissues in the path of the radiation beams. In addition, the only specificity for the tumour is conferred by the use of radiation beams which intersect at the tumour core, meaning that the tumour receives more radiation than the surrounding tissues. However, significant destruction of healthy tissues is unavoidable, limiting the dose of radiation that can be delivered and the effectiveness of the treatment. Chemotherapy remains the most commonly used treatment and typically involves giving patients large doses of cytotoxic drugs that circulate throughout the entire body, and which demonstrate a poor specificity for cancerous tissue. Consequently, they cause significant toxic effects elsewhere in the body. Common side effects include nausea, hair loss and suppression of the immune system. Professor Webb sums up this issue, which motivates his research, by saying: ‘Conventional chemotherapy suffers from the complications of adverse, off-target side effects. It is important to investigate and develop systemic treatment options that are less harmful to patient health in general’. Therefore, significant efforts have been devoted to developing new systemic therapies that are more targeted, reducing or eliminating side effects and increasing toxic effects at the tumour site. At the forefront of this research is an advanced suite of nanotechnologies, to permit for highly specific tumour targeting and controlled release of therapeutic drug payloads. Typically, this constitutes nanoparticles, introduced to the bloodstream, which are loaded with a drug and have the ability to passively accumulate in tumour tissues, due to the so called leaky vasculature inherent in most tumours, or are actively targeted to tumour cells. Of those types of nanoparticles that employ active targeting, many are decorated with tumourspecific antibodies to aid binding and uptake into tumour cells. Antibodies are specialised protein structures that can bind to a specific target antigen molecule with high specificity and are produced by the body to neutralise pathogens. Modern biotechnology provides a means to produce antibodies in the lab tailored to bind to antigens that are only expressed, or are more highly expressed, by cancer cells. Antibodies have been used as anti-cancer therapeutics in their own right. For example, Herceptin is an antibody therapeutic used against HER2, an antigen over-expressed on the surface of certain breast cancers. Nanoparticles decorated with tumour-specific antibodies selectively bind to and are absorbed by tumour cells, significantly enhancing the ability of their payload of drugs to produce toxic effects in the tumour itself and reducing the likelihood of drug exposure in healthy tissues. Nanoparticles have been designed using a range of biocompatible materials, including gold. Gold is a particularly useful material for nanoparticle design, as gold nanoparticle surfaces are highly amenable to chemical conjugation of proteins such as antibodies. In addition, gold nanoparticles demonstrate low toxicity, a large surface-to-volume ratio and are extremely small (10-20,000 would fit across the width of a human hair) thus enabling them to readily penetrate leaky tumour vessels to accumulate preferentially in cancerous tissues, all of which render them ideal as systemic anti-cancer carrier vehicles.
‘It struck me that applying nanotechnology was a great way to overcome the shortcomings of an older but promising technology like boron neutron capture’
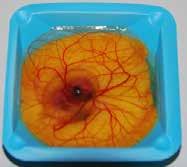
10 day ex ovo chick embryo
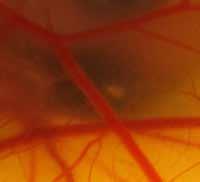
Bolus of human cancer cells injected into CAM
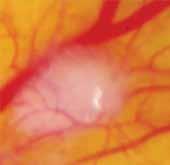
Tumour xenograft 6 days later
Boron neutron capture
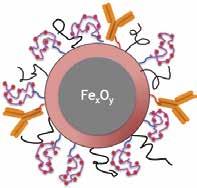
Nanoparticle construct. Orange – targeting antibody; purple – synthetic peptide with boron 10 molecules attached (red balls); m-PEG (black squiggly lines) stealth coating agent.
One clever approach to targeting cancerous tissues while avoiding damage to healthy tissues is boron neutron capture. The method is sophisticated, as it uses two treatments that are much less harmful to healthy tissues than many conventional cancer therapies, and which only become toxic when they combine inside the tumour. The binary technique involves the intravenous delivery of a non-radioactive isotope, boron-10, into the bloodstream of a cancer patient. As the boron-10 is not radioactive, it causes little or no damage to the tissues of the body. The boron-10 permeates the cells of the tumour. Tumours tend to have leaky blood vessels, resulting in more boron-10 accumulating there, than in healthy tissues. The tumour is then irradiated, using a modified nuclear reactor, to create a specialised type of radiation, which consists of a beam of low-energy thermal neutrons. The boron-10 in the tumour captures these neutrons, and undergoes nuclear fission to yield high energy alpha particles and lithium atoms. which by virtue of their large mass and short path length destroy only the tumour cells they occur in, resulting in the destruction of the tumour with little collateral damage. Healthy tissues surrounding the tumour and elsewhere in the body contain less boron-10, and are largely unaffected by the neutron bombardment at clinically relevant doses.
While this is an elegant solution to achieve tumour specificity, unfortunately, in practice the technique has been hampered by poor or unpredictable uptake of boron-10 into cancer tissues, resulting in insufficient tumour toxicity under neutron irradiation. In fact, to date, the technique has only been applied with a measure of success in brain tumours, which allow for the uptake of sufficient quantities of boron-10 to be successfully treated with neutron bombardment. The technique also faces another difficulty, whereby it has been impossible to adequately monitor boron-10 levels and distribution in the body, so that optimal dosage and timing of neutron bombardment can be achieved to maximize therapeutic effects. Consequently, while the method underwent extensive trials in patients it has been largely abandoned since the 1990s, due to poor therapeutic outcomes and difficulties in optimising the process.
Putting it all together – Professor Webb’s approach
Professor Webb’s team of undergraduates have considered the challenges facing boron neutron capture as a promising method of cancer therapy and have applied modern The bright green spots indicate multiple human tumour xenografts stained for blood vessels. nano- and biotechnological methods to resurrect this once neglected technique. They have developed tumourspecific antibody-decorated gold nanoparticles, loaded with boron-10 enriched compounds. These should allow more boron-10 to be absorbed by the tumour cells, compared with previously used boron-10 compounds that have been tried as delivery agents in prior attempts at boron neutron capture cancer therapy. Not only this, but the team have also designed these nanoparticles with a superparamagnetic core, to allow them to be visualised using magnetic resonance imaging (MRI). This means that the distribution and loading of the particles in a tumour can be visualised and assessed, permitting for optimal timing of the neutron beam to achieve maximal effects.
Given that these nanoparticles can preferentially bind to tumour cells and can be visualised with non-invasive imaging such as MRI, they could also potentially be used for diagnostic applications such as mapping and identifying cancerous tissue. This combination of diagnostic and therapeutic applications in one deliverable therapeutic means that the particles can be designated as ‘theranostic’. Professor Webb tells Scientia: ‘It struck me that applying nanotechnology was a great way to overcome the shortcomings of an older but promising technology like boron neutron capture. Nanoparticles have proven to be attractive platforms as theranostic agents in oncology by coupling high concentration targeting of therapeutics with imaging. For binary treatment modalities such as boron neutron capture, this is an ideal combination’.
The chick embryo chorioallantoic membrane assay
The team have applied a novel in vivo assay to test the potential of their nanoparticle system to specifically seek out and bind to cancer cells. Most research groups use immune deficient mice when examining the ability of a developed therapeutic to produce a cytotoxic effect in a human tumour, or even at an early proof-of-concept stage, where the ability of the therapeutic to bind to a tumour is assessed. The tumour is formed by simple injection of human cancer cells beneath the skin of the mouse, and different cancers can be studied by delivering cells derived from different types of cancer. Once the tumour is established and has acquired a blood supply, the therapeutic can then be delivered, and effects such as changes in the size of the tumour, animal mortality and determining whether the therapeutic has localised to the tumour, can all be assessed. However, such mammalian models are highly expensive. The mice must be immune deficient so that they do not reject the introduced human cells (growing cells or tissue of a different species in an organism is known as a xenograft) and consequently need to be housed under stringent pathogen-free conditions. In addition, such studies also raise ethical concerns, especially for early stage proof-of-concept projects, where preliminary data are collected and evaluated, concerning completely untested treatments. Professor Webb recognised that another technique, the chick embryo chorioallantoic membrane (CAM) assay, could be used for early-stage assessments of nanoparticle behaviour in tumour xenograft, as a viable alternative to an immune deficient mouse tumour model. His research team have employed this technique as a platform to assess nanoparticle uptake and targeting specificity in xenografts of pancreatic tumours. This approach is ethically preferable for early-stage work as it where mammalian animals like mice, are substituted with 4-day-old chick embryos which possess only limited sentience. Additionally the assay is simple to perform. Fertilised chicken eggs are cracked and the contents are deposited in a dish, which is cultured in a humid incubator. The highly vascularised CAM is exposed, and samples of human cancer cells can be injected, grown and visualised under the membrane, without the risk of immunological attack. The injected cancer cells become highly vascularised and form discreet tumours in the membrane tissue within 2–3 days. Nanoparticles can be injected into a vessel near the tumour xenograft and nanoparticle uptake and binding can easily be measured.
‘Nanoparticles have proven to be attractive platforms as theranostic agents in oncology by coupling high concentration targeting of therapeutics with imaging’
To date, the team have used the platform to investigate the binding of their nanoparticles to pancreatic adenocarcinoma (PDAC) cells. They cultured two different PDAC cell lines in the fertilised egg membranes, both of which express different biomarkers, to assess the specificity of their antibody-decorated nanoparticles.
Future work for the team.
The results to date have been extremely encouraging. The team have demonstrated that their antibody-decorated gold nanoparticles can undergo highly specific targeting to tumour-cell biomarkers and subsequent cellular uptake in vivo. The next steps are to begin to test the system as a whole, in its potential to successfully and specifically deliver sufficient amounts of boron-10 to tumours in vivo and determine if the delivered boron-10 can produce cell-destroying nuclear reactions when bombarded with neutrons. This will involve using MRI to visualise the nanoparticles in the CAM xenografts, and irradiating them at the nearby Massachusetts Institute of Technology nuclear reactor, where boron neutron capture was first pioneered for clinical use in the 1990s. Professor Webb is keen to emphasise that this research is a highly collaborative effort, and the hard work and dedication of both his students and faculty collaborators has made these innovative approaches possible.
Meet the researcher
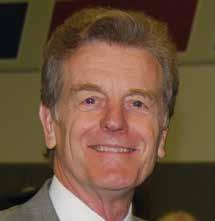
Professor Andrew Webb
Professor of Biological Sciences
Department of Biological Sciences
Wellesley College, MA 02481, USA.
Professor Andrew Webb is a Professor in the Department of Biological Sciences at Wellesley College. After graduating from Southampton University in the UK with a bachelor’s degree in Zoology and PhD in Developmental Biology, Professor Webb took a post-doctoral fellowship at Purdue University, Indiana USA. He was appointed to the faculty of Wellesley College just outside Boston in 1975 where he has taught courses in Organismal & Developmental Biology, Cell & Molecular Biology, Genetics, Comparative Anatomy, Bioinformatics & Cancer Genomics. His undergraduate research group was instrumental in cloning several human genes of medical interest in the 1980s (e.g. interleukin-1, parathyroid hormone-related peptide, plasminogen activator inhibitor-2), before moving to the development of nanoparticles as theranostic agents in oncology. He has authored over 50 peer-reviewed articles, is the inventor on over a dozen biotechnology patents and has served as a consultant and expert witness for numerous pharmaceutical and biotechnology companies.
CONTACT
T: (+1) 781 283 3115
W: http://www.wellesley.edu/biology/faculty/webba
KEY COLLABORATORS
Dr Nolan Flynn, Department of Chemistry, Wellesley College, USA
Dr Nancy Kolodny, Department of Chemistry, Wellesley College, USA
Dr Kent Riley (deceased), Massachusetts General Hospital, Boston, USA
FUNDING
National Cancer Institute, NIH
Staley, Fiske & Wellesley College Faculty Research Awards
REFERENCES
Webb, A.C., Choi, R., Sun, S, Flynn, N., Kolodny, N.H. & Riley, K. (2012), Resurrection of boron neutron capture therapy in an era of nano-oncology, 20th Ann. Cancer Res. Inst. Internat. Sym. Cancer Immunotherapy, New York, Oct. 1–3.
Nagalla, R., Kolodny, N.H., Webb, A.C., Flynn, N. & Liao, A. (2013), Functionalizing silica-coated iron oxide nanovehicles for imaging and targeted cancer therapeutics, Abstracts of Papers, 244th ACS National Meeting; American Chemical Society, Washington, DC., Sept. 8–12.
Musetti, S., Cheema, A., Flynn, N., Kolodny, N. & Webb, A.C. (2015), Investigating the integrity of novel nano-vehicles for treating pancreatic cancer, Abstracts of Papers, 249th ACS National Meeting; American Chemical Society, Denver, CO., Mar. 22–26.
Pollack, I., Verma, K., Begg, B., Truong, T., Natarajan, H. & Webb, A. (2016), The chick chorioallantoic membrane (CAM) as an early stage in vivo model for assaying the specificity of nanoparticle targeting to tumors, Nanomedicine (submitted). pii:S0304–3835(15), 00771–5.
