Professors Roger Koeppe II and Denise Greathouse at the University of Arkansas design and study peptides that interact with model biological lipid-bilayer membranes. They are interested in understanding the protein-lipid molecular interactions that are responsible for the biological function of membrane proteins, and they also aim to gain a better understanding of the differences in ionisation behaviour of functional groups in membrane versus aqueous proteins.
The Importance of peptide-membrane interactions
The cell membrane is a complex, selectively permeable, bilayer structure that separates the interior of cells from the outside environment. One feature of the cell membrane that is essential for numerous biological processes is its ability to interact with proteins. The building blocks of proteins are the 20 different amino acids, each having unique chemical characteristics such as size, solubility preference, and charge. Membrane interacting peptides are small proteins made up of less than 50 amino acids. They exhibit a wide range of biological activities and are involved in a vast array of cellular functions, such as antimicrobial defence mechanisms, membrane fusion, viral translocation and the transport of therapeutic compounds. Thus, due to their potential biomedical applications, membrane-active peptides are a major focus of much current research.
When peptide-membrane interactions occur, both the peptide and the membrane can undergo a series of conformational and/ or structural changes. Researchers use both theoretical and experimental studies to gain insights into peptide-membrane interactions. This is a highly challenging field of research, with the overall goal of achieving a better understanding of the relationship between the amino acid composition of the peptide and the manner in which the peptide interacts with the membrane. Many of the molecular details of these processes, however, remain elusive. This is where the work of Professors Roger Koeppe II and Denise Greathouse comes into play. Their research employs experimental methods to focus on understanding the molecular mechanisms behind the specific interactions that determine how peptides that span and anchor to membranes interact with lipids and adjust their geometry in response to changes in the environment. The experimental results in turn can help to advance theoretical understanding and computational predictions.
As part of their research, the pair is interested in membrane-peptide interactions and how this knowledge can be used to treat resistant strains of bacteria. ‘Membrane-active peptides and proteins are vital to many physiological processes, including signal transduction and ion conduction, and many have anti-microbial properties,’ Professor Greathouse explains. There is an increasing occurrence of multi-drug resistant bacteria, in both the healthcare and community settings. Currently, there is a scarcity of new antimicrobial drugs to fight these bugs, and this poses a great threat to public health. Our first line of defence when our bodies are presented with a new pathogen, like one of these drug-resistant bacteria, is the innate immune system. This aspect of our immunity might be a rich source of prototype molecules (peptides) for the development of new anti-infection agents. Amongst these peptides are the mammalian lactoferricin peptides, derived from lactoferrin proteins after natural proteolytic digestion and rich in the amino acids tryptophan (Trp) and arginine (Arg). The research being pursued by Professors Koeppe and Greathouse and their team aims to understand the membrane interactions and mechanisms of action of peptides within the lactoferricin family.
Subtle peptide changes lead to great functional consequences
Amino acids are the building blocks of proteins, and peptides can be simply described as short proteins. The properties of amino acids contribute to the overall function of proteins and peptides. Lysine (Lys) and arginine (Arg) are both basic amino acids, and they possess important functions involved in membrane protein activity. These amino acids can sense membrane voltages and play roles in the actions of antimicrobial, toxin and cell-penetrating peptides. One key feature of amino acids that can be used to regulate protein function is the ionisation state. Atoms or molecules can become ionised by acquiring charge through the gain or loss of protons (hydrogen ions). When a peptide is trapped within the lipid-bilayer of the cell membrane, it is difficult to decipher the ionisation states of the individual functional groups. Professors Koeppe and Greathouse and their colleagues have addressed this issue for the functional components of the amino acids Lys and Arg in designed transmembrane peptide helices. In their method, they introduced a “guest” Lys or Arg residue at a certain position in a “host” membrane-spanning peptide. They found that in bilayer membranes at a low pH, when Lys is positively charged as a result of binding to a hydrogen ion, the Lys residues in the peptides behaved similarly to arginine. However, when the pH was elevated above pH 7, they observed that the Lys titrated (lost its charge) in the bilayer membrane, whereas the Arg remained charged. The researchers assessed the pH-dependent changes that occurred in the ‘tilt’ of the transmembrane peptide helix when each single Lys was titrated. They discovered that a buried charged Lys at certain positions within the transmembrane helix, like Arg, will cause the peptide tilt to change in order to maximise access of the charge to the aqueous interface. If, however, the charged residue is ‘sandwiched’ between neighbouring aromatic rings of Trp residues, which block access of the charges to the aqueous interface, the transmembrane peptide will leave the lipid bilayer. ‘One of the important results from this research is that the pH at which ionisable amino acids titrate (the pKa) when they are located in a membrane environment is very different from their pKa in an aqueous environment. Professor Greathouse explains, ‘These findings have important implications for understanding the mechanisms of large membrane embedded proteins such as those comprising the photosynthetic reaction centre and voltage gated ion channels, and are providing key information to facilitate methods that involve molecular dynamics simulations.’
Professor Koeppe describes the relevance of this work for biomedical applications: ‘Better knowledge of these fundamental molecular properties will lead to a better understanding of the mechanisms by which key signalling proteins function in biological membranes. Gaining greater insight into molecular function in turn leads to a better understanding of healthy and diseased states and may offer clues for new treatment options.’
‘I remain fascinated by molecular function and the enormous variety of protein properties that can be realised using only twenty fundamental amino-acid building blocks’ – Professor Koeppe
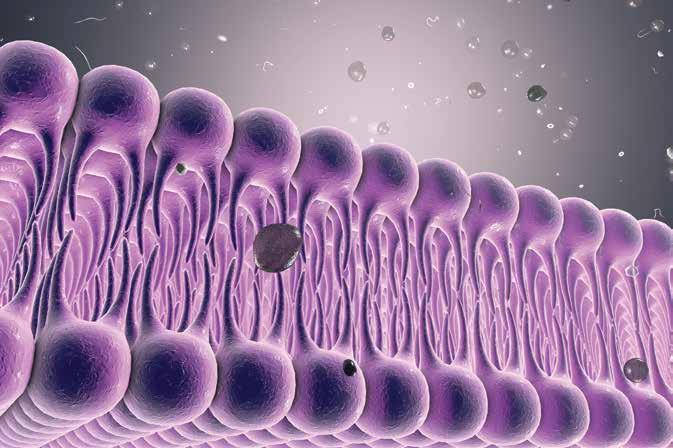
Aromatic amino acids alter the orientation of peptides in the lipid bilayer
In their model peptides, Professors Koeppe and Greathouse include a core leucine−alanine sequence to promote the formation of a helical conformation and enhance the sensitivity of the peptide to the membrane thickness due to any hydrophobic mismatch between peptide and lipid. Aromatic amino acids are often located at the ends of transmembrane alpha helices of integral membrane proteins. Because of their preference for locations within the membrane−water interface of the lipid bilayer, the aromatic residues tryptophan (Trp), tyrosine (Tyr) and sometimes phenylalanine (Phe) might function as anchors to help stabilise the transmembrane orientation. Therefore, the team compared the influence of Trp, Tyr and Phe amino acid residues at the membrane− water interface of the lipid bilayer upon the properties of tilted helical transmembrane peptides.
The team hypothesised that by altering the identities or positions of the aromatic anchors that surround the core sequence of a model peptide, the effects of these substitutions on the orientations and dynamics of the transmembrane helices could be investigated, and the results translated to better understand the behaviour of helices in membrane proteins. They discovered that when Trp, Tyr or Phe are replaced at the same position, one at a time within a model peptide, the peptide has the same average tilt and exhibits similar dynamics in the bilayer membranes. However, when two Tyr anchors are present, the model peptide exhibits more dynamic averaging and less responsiveness to the bilayer thickness than when two Phe anchors are present. Though Phe and Tyr are similar, it is notable that Phe lacks the hydrogen-bonding ability that is a crucial property of Tyr. These results suggest that subtle changes in the type of aromatic amino acid at the membrane-water interface can have dramatic effects on peptide helix rotation and dynamics and on the lipid membrane thickness. These effects may be important mechanisms that proteins use to regulate certain biological functions. The team concluded from this study that in the absence of other functional groups, aromatic residues at the membrane−water interface of the lipid bilayer determine the preferred orientations and dynamics of membrane-spanning peptides, which suggests possibilities for the rotational and dynamic control of membrane protein function.
A new way to study peptide helix stabilisation
The research conducted by Professors Koeppe and Greathouse has contributed to the notion that the dynamic properties of similar transmembrane helices can differ widely. While the exact mechanisms that direct the orientation or extent of helix dynamic averaging are not fully understood, it is clear that the type and location of amino acids, with slightly different properties, within the helix can have dramatic effects on the way the peptides interact with membranes, and therefore are important for defining the functional properties of membrane proteins. One possibility is that having ‘too many’ Trp or Tyr residues at the membrane−water interface of the lipid bilayer might correlate with high levels of dynamic averaging. The team sought to address additional factors, aside from the role of the Trp or Tyr aromatic rings at the membrane−water interface of the lipid bilayer, that could be responsible for stabilising the preferred orientations and limiting the dynamics of single transmembrane helices. To do this, they examined whether the amino acids at the ends of the peptide, outside the anchoring aromatic amino acids, might be unwound (frayed) when they are in the bilayer membrane environment. Indeed, the team observed substantial unwinding or fraying of the amino acids at the ends of several tilted helices spanning the membranes of the lipid bilayers.
The observation of helix fraying led to speculation that hydrogen bonding between the unwound end segments and the membrane might be an important mechanism for reducing peptide dynamics and stabilising transmembrane peptides in lipid bilayers. As an example, it is already known that helix fraying is a mechanism used by multi-span proteins, such as G protein-coupled receptors, to adapt to a decrease in the hydrophobic thickness of the surrounding membrane. The model peptide–lipid systems designed by Professors Koeppe and Greathouse provide a novel way to address the intrinsic nature and potential significance of transmembrane helix fraying for membrane protein function.
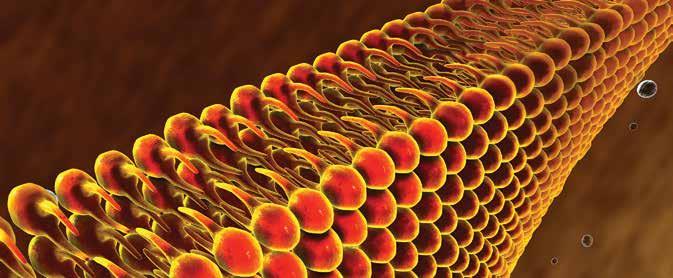
‘One important result from our research is that the pH at which ionisable amino acids titrate when they are located in a membrane environment can be very different from when they are in water’ – Professor Greathouse
Future Studies
The team is characterising the transitions of the amino acids lysine (Lys) and histidine (His) between neutral and positively charged states in several different lipid bilayer membranes. ‘Next we want to characterise the ionisation behaviour of glutamic acid (Glu) and aspartic acid (Asp) as they transition between neutral and negatively charged states in similar bilayer membranes.’ Professor Koeppe explains. ‘We also would like to investigate the influence of cholesterol on the ionisation properties that we measure.’
Meet the researchers
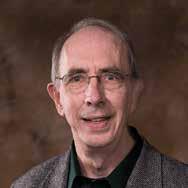
Professor Roger Koeppe II
Distinguished Professor
Department of Chemistry and Biochemistry
J. William Fulbright College of Arts and Sciences
University of Arkansas
Professor Roger Koeppe II is a distinguished professor in the Department of Chemistry and Biochemistry in the J. William Fulbright College of Arts and Sciences at the University of Arkansas. During his undergraduate studies in mathematics and chemistry at Haverford College, Professor Koeppe became intrigued with quantitative aspects of biological chemistry. From here, he then went on to receive his PhD from the California Institute of Technology, and did several years of postdoctoral work in Stanford. He has been recognised with an NSF Predoctoral Fellowship, an NIH Research Career Development Award, a Fulbright Fellowship (Netherlands, 1992), the Arkansas Alumni Research Award and the Fulbright College Master Researcher Award.
CONTACT
E: rk2@uark.edu
T: (+1) 479 575 4976
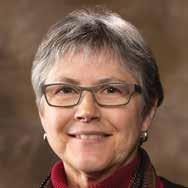
Professor Denise Greathouse
Research Associate Professor
Department of Chemistry and Biochemistry
J. William Fulbright College of Arts and Sciences
University of Arkansas
Professor Denise Greathouse is an Associate Professor in the Department of Chemistry and Biochemistry in the J. William Fulbright College of Arts and Sciences at the University of Arkansas. Professor Greathouse’s journey to becoming a peptide chemist began when she was a lab manager at the University of Wyoming, using one of the first fully automated commercial solid phase peptide synthesisers, the Applied Biosystems 430A. Her research now focuses on the synthesis of peptides designed to interact with cell membranes. Professor Greathouse has been recognised with the Faculty Gold Medal for undergraduate mentoring at the University of Arkansas.
CONTACT
T: (+) 479 575 7471
KEY COLLABORATORS
Professor Olaf S Andersen, Weill Cornell Medical College, New York, USA
Professor Alan Grossfield, University of Rochester Medical Center, USA
Professor J Antoinette Killian, University of Utrecht, the Netherlands
Professor Stanley J Opella, University of California, San Diego, USA
Professor Mark S P Sansom, University of Oxford, UK
FUNDING
National Science Foundation – grant no. MCB1327611
Arkansas Biosciences Institute
REFERENCES
A Mortazavi, V Rajagopalan, KA Sparks, DV Greathouse and RE Koeppe II, Juxta-terminal Helix Unwinding as a Stabilizing Factor to Modulate the Dynamics of Transmembrane Helices, ChemBioChem, 2016, 17, 462–465.
KA Sparks, NJ Gleason, R Gist, R Langston, DV Greathouse and RE Koeppe II, Comparisons of Interfacial Phe, Tyr, and Trp Residues as Determinants of Orientation and Dynamics for GWALP Transmembrane Peptides, Biochemistry, 2014, 53, 3637−3645.
NJ Gleason, VV Vostrikov, DV Greathouse and RE Koeppe II, Buried lysine, but not arginine, titrates and alters transmembrane helix tilt, PNAS, 2013, 1692–1695.
