Dr Michael Parker – Dr Christopher Jeynes | Explaining Fundamental Reality (using the changing entropy)
Dr Michael Parker (Lexden Technologies) and Dr Christopher Jeynes (independent scholar) apply basic principles of thermodynamics to the topical issue of beta-decay bringing us an entirely new understanding of Time and Reality.
How Much Do We Really Know?
What is the world made of? How did we get here? Surely we have answers to these seemingly basic questions? After all, we know when the Big Bang took place and that all the elements were made in the stars (and pretty much how the stars were made, too). Moreover, our lives have been transformed by technological advances: GPS, satellite views of Earth, mobile phones, and the internet – wonders unheard of even a generation ago.
But it seems that the more we find out, the more we realise we don’t actually know. Quantum mechanics (QM; the study of atoms, radioactivity, and so on) is very well-known and successful, as is general relativity (GR: black holes, galaxies, and so on). If we dig a little deeper, though, we realise that we still haven’t worked out quantum gravity (QM+GR) properly. Then add to this the ‘small’ problem of Time. Both QM and GR are time-reversible (they both work just fine if you run the clocks backwards), but we know that life is not reversible (we live first and die afterwards, not the other way round). Something is clearly missing from our current explanations.
New Answers to Old Questions
In 2023, Dr Michael Parker (Lexden Technologies) and Dr Chris Jeynes (independent scholar) made giant steps in answering these deep questions by accepting the Second Law of Thermodynamics (one of the great general principles of physical science) as fundamental, and not derived somehow from QM or GR. Note that the Second Law fixes the ‘Arrow of Time’: things go from the past to the future, and revisiting the past is forbidden. Therefore, neither QM nor GR (nor both together) can be the whole truth since (being reversible) they do not properly recognise the prominence of irreversibility in the real world.
Usually, physicists treat these ‘fundamental theories’ (as currently thought: the time-reversible ones, QM and GR) as basic, and explain real-world behaviour by cunning tweaks to their basic behaviour. Often, this works surprisingly well. Except when it doesn’t (and then we give up). So, it was a tremendous advance in 2023 when we (Parker & Jeynes) showed how reversible and irreversible behaviours can actually be described in a unified way. But this is not all …
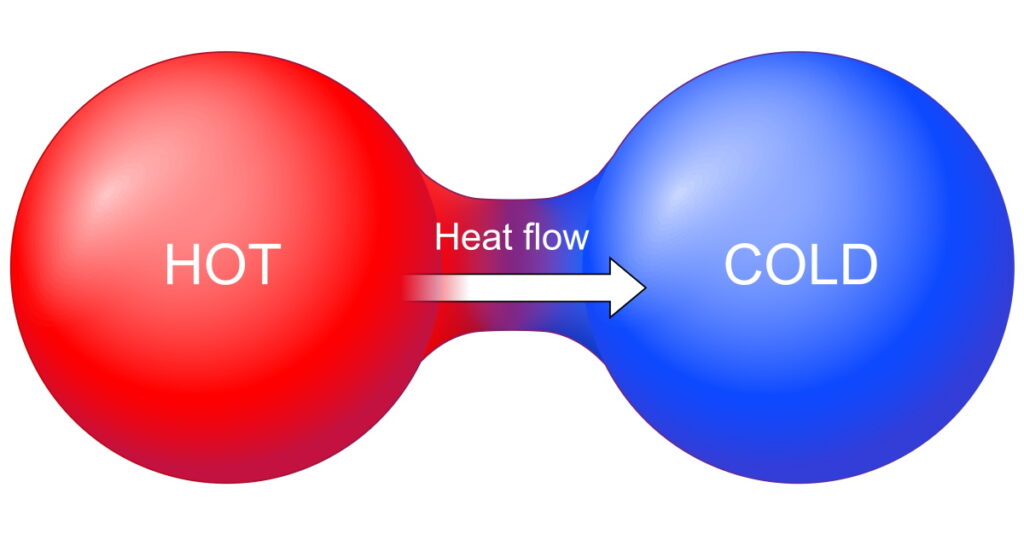
The Second Law of Thermodynamics
Hot things cool down
A New Understanding of Time (and Thermodynamics)
We only know that time is passing by watching things change, and the most basic sort of change is the sub-atomic beta decay process (see the ‘beta decay’ and the ‘αβγ’ text boxes). However, we (Parker & Jeynes) showed in 2023 how beta decay times can be obtained from our new thermodynamics (Quantitative Geometrical Thermodynamics ‘QGT’). This is important because if the Second Law is fundamental, then there should also be a fundamental description of how change happens.
Beta-decay rates are needed to implement realistic models of stellar evolution, and can be calculated from quantum mechanics, but these calculations are not sufficiently reliable to be useful for isotopes that can’t be measured – which is most of them. Moreover, it turns out to be really tricky to measure the decay rate for even the simplest process – that of the free neutron spontaneously turning into (or decaying to) a proton.
However, by using our new methods of thermodynamics (QGT, which treats the Second Law as fundamental) we have shown that we can calculate the lifetime of the free neutron in two lines of arithmetic. The time behaviour comes from the Planck-Einstein relation (which says that the energy quantum of the photon is proportional to the frequency) and our previous finding that energy and entropy production (the rate of creating entropy) are the same (sort of, looked at the right way). This is important since we all know that energy is conserved – there’s no such thing as a free lunch.
The Second Law states that entropy always increases; that is, any real system dissipates energy (creating entropy), and for systems in a Maximum Entropy (‘most probable’) state we have shown that entropy production is constant. Black holes are certainly Maximum Entropy entities, but they also necessarily grow. If they grow they create entropy, but this must be at a constant rate because entropy production is also a conserved quantity.
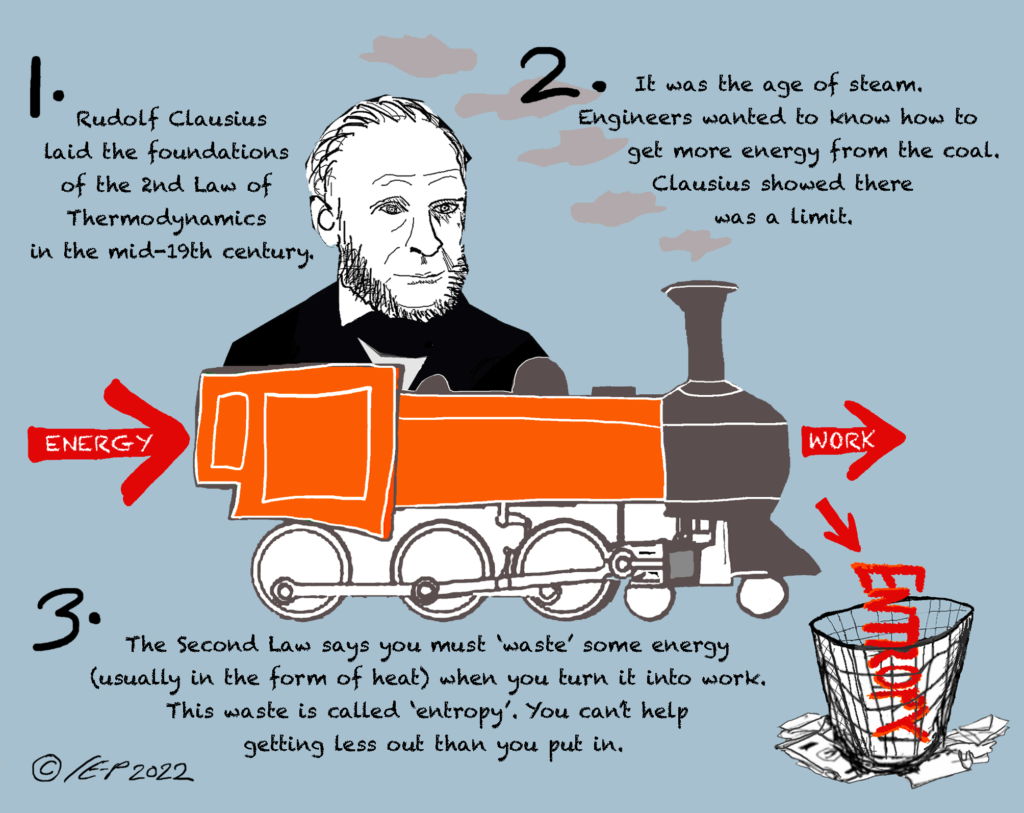
The Second Law of Thermodynamics
No such thing as a free lunch
Credit: Christine Evans-Pughe, www.howandwhy.com
Calculating Entropy from Geometry
We also need to know that the entropy of a structure may be calculated quantitatively simply from its geometry: we introduced QGT in 2019, remarkably allowing us to calculate the size of the alpha particle, without any quantum mechanics, using the Bekenstein-Hawking equation (famous for being derived by Jacob Bekenstein in 1972 specifically to obtain the entropy of black holes, but derived independently by QGT). This shows that alpha particles are like black holes in some ways, which is a strange and challenging idea!
Conclusion (Time is Real)
So, what is fundamental? How do we know what is real? Jeynes and Parker argue that fundamental physics really does back up what everyone knows viscerally – that Time is real. The time reversibility of the so-called ‘fundamental’ equations of quantum mechanics and general relativity simply ignores the irreversibility of the world. It is the Second Law of Thermodynamics that is fundamental.
Beta decay
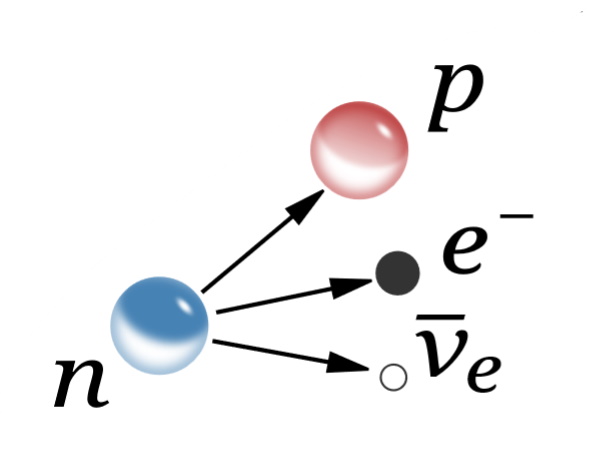
The neutron decays into a proton, an electron and an antineutrino, increasing the total entropy.
An atom’s nucleus contains protons and neutrons. When neutrons are in the nucleus, they are (usually) perfectly stable. But a neutron by itself (a so-called ‘free neutron’ n) doesn’t like it, and after a while ‘decays’ into a proton p, spitting out an electron e– and an electron antineutrino ν̅ e (the antimatter form of the neutrino). This is the beta decay process. Neutrons inside unstable nuclei (like 8Li) may also beta decay to protons (see the αβγ text box).
The ‘half-life’ of the free neutron is about 10 minutes, meaning that if you start with 1,000 free neutrons, after 10 minutes you will have approximately 500 left.
αβγ: Alpha, Beta, Gamma
The Big Bang
The famous ‘alphabetical paper’ (Alpher, Bethe & Gamov, The Origin of Chemical Elements, 1948) was the first to effectively propose the Big Bang (George Gamov was poking fun at Aristotle with the αβγ joke: Aristotle thought time was necessarily infinite). Alpha and beta (and later, gamma) particles were so-called by Ernest Rutherford because that was their order of discovery (1899 and 1903) when radioactivity was the latest new thing. The ‘alpha’ is a helium atom without its two electrons (Rutherford got the Nobel Prize in 1908 for proving this), the ‘beta’ is simply an electron, and gamma rays are photons much more energetic than X-rays (which were discovered by Wilhelm Röntgen in 1895).
The Geometry of Atoms
Atoms are made of a tiny central nucleus (positively charged) with an outer ‘shell’ of electrons (negatively charged). Nuclei are made of protons (positively charged) and neutrons (uncharged). Normally, one would expect ‘like charges to repel’, and therefore, nuclei as we have just described shouldn’t exist – but they do! It seems that the neutrons are a sort of glue. Atoms are neutral (uncharged) because there are always as many electrons as protons. But there may be more or less neutrons, resulting in ‘isotopes’. For example, you can get helium (He; two protons) with 1 or 2 neutrons, giving 3He or 4He. Similarly, you can get 6Li and 7Li (lithium with 3 protons but 3 or 4 neutrons), or 12C and 13C (carbon with 6 protons but 6 or 7 neutrons).
Isotopes (Mostly Unstable)
The thing is, thinking about isotopes of lithium (say), you can make 8Li by squeezing an extra neutron into 7Li, but it doesn’t like it, and after about 0.8 seconds (actually a very long time in nuclear physics!) it changes by beta decay to 8Be (which immediately disintegrates to two alphas). The ‘Chart of Nuclides’ shows all the nuclei that we know exist (however fleetingly: like 8Be, most are unstable). In stellar nucleosynthesis, all of these isotopes are made, and after some time only the (approximately) stable isotopes remain. Planets (such as ours, for instance) are made up mostly of stable isotopes (and the radioactive ones are very long-lived).
SHARE
DOWNLOAD E-BOOK
REFERENCE
https://doi.org/10.33548/SCIENTIA1034
MEET THE RESEARCHER
Dr Chris Jeynes
CPhys, CEng, CChem
Independent Scholar, Tredegar, Wales
Dr Chris Jeynes obtained his PhD ‘Carbonado and the diamond polishing process’ from the University of Bristol in 1981. He joined the University of Surrey by invitation, and became the Ion Beam Centre Liaison Fellow (1982–2017). He was appointed Professorial Research Fellow in 2015, Visiting Professor in 2020, and formally retired in 2023. He has an international reputation as a metrologist and for spectrometry (accurate ion beam analysis) with over 300 papers in the peer-reviewed literature. Dr Jeynes is a member of both the Royal Society of Chemistry and the Institute of Physics. He is a Chartered Physicist, a Chartered Engineer, and a Chartered Chemist.
CONTACT
W: https://www.surrey.ac.uk/people/chris-jeynes
Dr Michael C Parker
Senior Member IEEE, CEng
Lexden Technologies, Epsom, England
Dr Michael Parker obtained his PhD ‘Dynamic holograms for wavelength division multiplexing’ (applying novel disordered yet informationally-rich geometries to telecommunications technologies) from the University of Cambridge in 1996. He gained extensive experience in industry both as an engineer and researcher before taking up a Visiting Researcher role at the University of Essex in 2006. Dr Parker is currently the Technical Manager for both a large EU/EPSRC research project (SAMBAS), and also an Innovate UK project (QAssure) on the assurance of systems based on quantum information technology, with a focus on quantum key distribution. In addition to publishing more than 170 peer-reviewed articles, Dr Parker holds more than 20 patents arising from his research. Dr Parker is a member of the American Institute of Physics, Optica, and the Institution of Engineering & Technology. He is a Chartered Engineer.
CONTACT
E: mcpark@essex.ac.uk
W: https://www.essex.ac.uk/people/PARKE13104/Mike-Parker
REFERENCES AND FURTHER READING
C Jeynes, MC Parker, M Barker (2023), The Poetics of Physics, Philosophies 8(1), 3.
M Gorchtein, C-Y Seng (2023), The Standard Model Theory of Neutron Beta Decay, Universe 9, 422.
MC Parker, C Jeynes (2023a), ab initio thermodynamics calculation of beta decay rates, Annalen der Physik 535, 2300259.
MC Parker, C Jeynes (2023b), Relating a system’s Hamiltonian to its Entropy Production using a Complex-Time approach, Entropy 25, 629. Selected as an Editor’s Choice paper.
MC Parker, C Jeynes, WN Catford (2022), Halo Properties in Helium Nuclei from the Perspective of Geometrical Thermodynamics, Annalen der Physik 534, 2100278.
MC Parker, C Jeynes (2021a), A Relativistic Entropic Hamiltonian–Lagrangian Approach to the Entropy Production of Spiral Galaxies in Hyperbolic Spacetime, Universe 7, 325.
MC Parker, C Jeynes (2021b), Entropic Uncertainty Principle, Partition Function and Holographic Principle derived from Liouville’s Theorem, Physics Open 7, 100068.
MC Parker, C Jeynes (2019), Maximum Entropy (Most Likely) Double Helical and Double Logarithmic Spiral Trajectories in Space-Time, Scientific Reports 9, 10779.
REPUBLISH OUR ARTICLES
We encourage all formats of sharing and republishing of our articles. Whether you want to host on your website, publication or blog, we welcome this. Find out more
Creative Commons Licence (CC BY 4.0)
This work is licensed under a Creative Commons Attribution 4.0 International License.
What does this mean?
Share: You can copy and redistribute the material in any medium or format
Adapt: You can change, and build upon the material for any purpose, even commercially.
Credit: You must give appropriate credit, provide a link to the license, and indicate if changes were made.
SUBSCRIBE NOW
Follow Us
MORE ARTICLES YOU MAY LIKE
Probing Electron Dynamics in the Ultrafast Regime
In the atoms that make up the matter around us, negatively charged particles called electrons have properties such as spin and orbital angular momentum. Researchers at Martin Luther University Halle-Wittenberg have developed a theoretical framework which allows them to simulate the dynamics of the spin and orbital angular momentum of electrons in materials when probed with an ultrafast laser pulse. Using this framework, they are able to simulate different materials and improve our understanding of dynamics on an atomic scale.
Detecting Waves and Particles Around the World – and Beyond!
The magnetic field around our planet, along with unique radioactive decay processes, can cause the generation of Alfvén waves. Dr Gerald Smith of Positronics Research LLC has been researching how we can observe these waves and the unique atoms formed in these processes. By looking at data locally and how these particle events are represented at telescopes and monitoring systems around the globe, Dr Smith observes their impacts and points to their potential in future interplanetary exploration.
Dr Jens Holger Rindel | The Acoustics of Open-Air Theatres: Why Traditional Parameters Don’t Apply
When you sit in an ancient Greek theatre, marvelling at how clearly you can hear performers even in the back rows without microphones, you’re experiencing acoustic engineering that predates modern science by millennia. According to new research, many of the standard methods acousticians use for concert halls are ineffective for open-air venues. Dr Jens Holger Rindel of Odeon A/S in Denmark has demonstrated why we need different acoustical parameters to evaluate open-air theatres properly. His work challenges the conventional wisdom of applying indoor concert hall metrics to outdoor venues and proposes new approaches specifically tailored to spaces where the sky forms the ceiling.
Calculating Steering Friction: Essential Engineering for Military Aircraft Safety
The safe operation of military training aircraft depends on precise engineering calculations that most of us never consider. Dr Bogdan Adrian Nicolin and Dr Ilie Nicolin from the National Institute for Aerospace Research in Romania have developed sophisticated methods to calculate the steering friction moment in military aircraft. This critical engineering parameter ensures that pilots can safely control aircraft during taxiing, take-off, and landing – making their work essential for both pilot training and flight safety.