Dr Nancy Chabot | Dr Carolyn Ernst | Ariel Deutsch – Icy Discoveries on Our Innermost Planet
The location of water in our solar system may hold the key to understanding how the planets evolved, and indicate other potential places to find life away from Earth. Dr Nancy Chabot and Dr Carolyn Ernst of Johns Hopkins University Applied Physics Laboratory, and Ariel Deutsch at Brown University, use data from NASA’s MESSENGER mission to understand how much ice exists on Mercury and how it may have arrived there.
The Search for Water
The question of whether we are alone in the Universe has fuelled humanity’s curiosity with space since we first looked up to the stars. From our perspective, Earth is the perfect habitable planet – it has a rocky surface with an atmosphere and liquid water to support life. By looking for evidence of water on other planets, scientists can start to understand how our solar system evolved and find clues for where to search for life in the wider universe.
In the early 1990s, radar images of Mercury’s surface taken from Earth identified highly reflective radar-bright areas representing deposits of ice. Dr Nancy Chabot and Dr Carolyn Ernst of Johns Hopkins University Applied Physics Laboratory, and Ariel Deutsch at Brown University study the details of ice on Mercury using data collected from NASA’s MErcury Surface, Space ENvironment, GEochemistry, and Ranging (MESSENGER) spacecraft.
NASA’s MESSENGER Mission
The MESSENGER mission was the first to visit Mercury since the Mariner 10 flybys in the mid-1970s, and the first mission ever to orbit the planet. MESSENGER orbited the planet between 2011 and 2015, providing an in-depth look at this hostile world. Mercury is the closest planet to the Sun, and experiences temperatures of up to 430°C. These extremely high temperatures, combined with Mercury’s lack of atmosphere, would normally mean that no water could condense on the planet’s surface.
Mercury rotates almost vertically on its axis. Mercury’s vertical rotation means that locations at the planet’s cratered poles are permanently in shade. In this shade, where direct sunlight never reaches, the temperatures can be very low, reaching −160°C where ice can be stable.
The MESSENGER spacecraft was equipped with a narrow-angle camera and a wide-angle filtered camera, and the combined instrument was called the Mercury Dual Imaging System (MDIS). This camera could image the surface of Mercury, including within the permanently shadowed polar regions. ‘We obtained the first images that successfully revealed what the surfaces of the ice deposits looked like,’ recalls Dr Chabot. ‘This was a really challenging observation, as we were trying to take images of surfaces that are always in shadow! We used settings on MESSENGER’s camera that let the extremely low levels of light that were reflected into the area from nearby crater walls and other surface features provide enough light to image the surface.’
In a study led by Deutsch, the team utilised over 16,000 images of Mercury’s north pole area to map the locations of permanent shadow, and combined these images taken by MDIS with MESSENGER’s Mercury Laser Altimeter (MLA) measurements. MESSENGER’s MLA measured the amount of time it takes for a laser pulse to travel to the surface and reflect back again, providing key information about the topography of the planet.
The team confirmed that the water ice signals detected by the Earth-based radar are located in the shadowed craters at the north pole. They then used MDIS images to see within the shadowed craters, revealing images showing that much of the ice is covered with an insulating layer of dark material and not loose rock. The dark material is speculated to be organic-rich volatile compounds. There have been various calculated estimates of the ice thickness, but the current best estimate of its maximum thickness is 15 metres. ‘Data from NASA’s MESSENGER mission, the first spacecraft to ever orbit the planet Mercury, have revolutionised our understanding of Mercury, including the ice in its polar regions,’ states Deutsch.
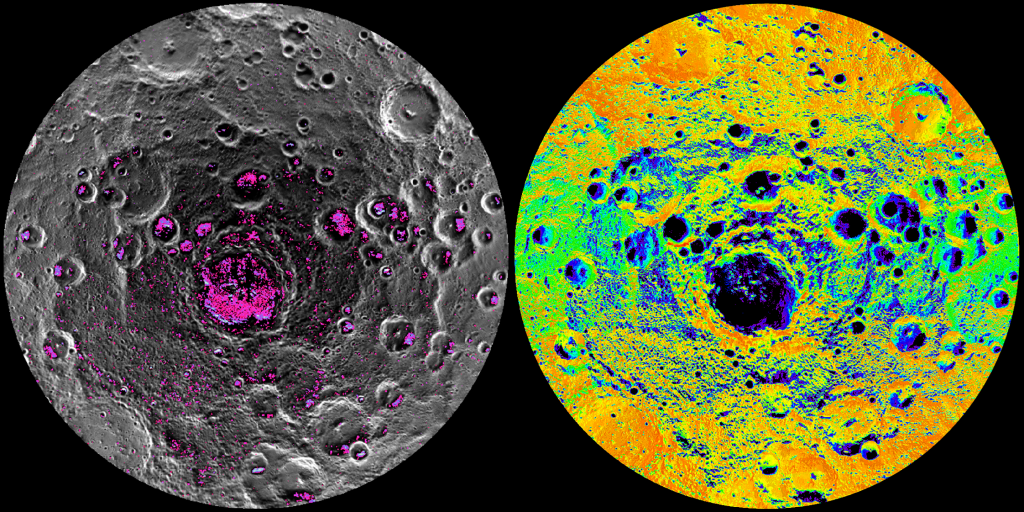
Mercury’s south polar region, with Arecibo radar data in pink indicating the locations of ice, and a colourised map of illumination conditions, showing regions of permanent shadow in black
Detailed Features
During the final year of its mission, the orbit of the MESSENGER spacecraft brought it closer to Mercury’s surface than before, reaching altitudes below 200 kilometres across the north polar region. This provided the team with an opportunity to gather more detailed images of the shadowed craters and ice deposits.
When the team analysed the MDIS data, they discovered that the ice deposits are not uniform, but rather have variations in their surface brightness. They noticed that there can even be quite a lot of variation within the same crater, such as the Desprez crater. At 47 kilometres wide, this is one of the largest shadowed craters imaged during MESSENGER’s low altitude orbit.
The team found that the variations in brightness matched the modelled variations in temperature across the Desprez crater, suggesting that the hypothesised organic compounds covering the ice varied with the location’s maximum surface temperature. At room temperature on Earth, these types of organic compounds will rapidly evaporate. However, under the extreme cold conditions in the shaded craters on Mercury, these compounds are stable and can act as an insulating cover on the water ice.
Comparing North and South Poles
NASA’s MESSENGER mission was able to provide high-resolution images of Mercury’s north pole due to the spacecraft’s eccentric orbit. This orbit meant that MESSENGER passed over the south pole at a far higher altitude – over 10,000 kilometres above the planet’s surface. While this meant that MESSENGER’s MLA instrument could not take measurements over the south pole, the mission still provided MDIS images for the team to analyse. These were a combination of standard and long exposure images that covered a large area of the south pole.
The team combined these images with new radar observations from the Arecibo Observatory in Puerto Rico to study the shadowed craters of Mercury’s south pole. They found that the locations of permanently shadowed craters in the south pole matched the locations of radar-bright deposits observed from Earth. There is around double the area of radar-bright deposits in permanently shadowed areas at the south pole compared to the north pole.
‘Our continuing analysis of Mercury’s ice deposits suggests that Mercury’s ice is fairly young and perhaps delivered by a large comet or asteroid impacting Mercury within the last tens of millions of years or so.’
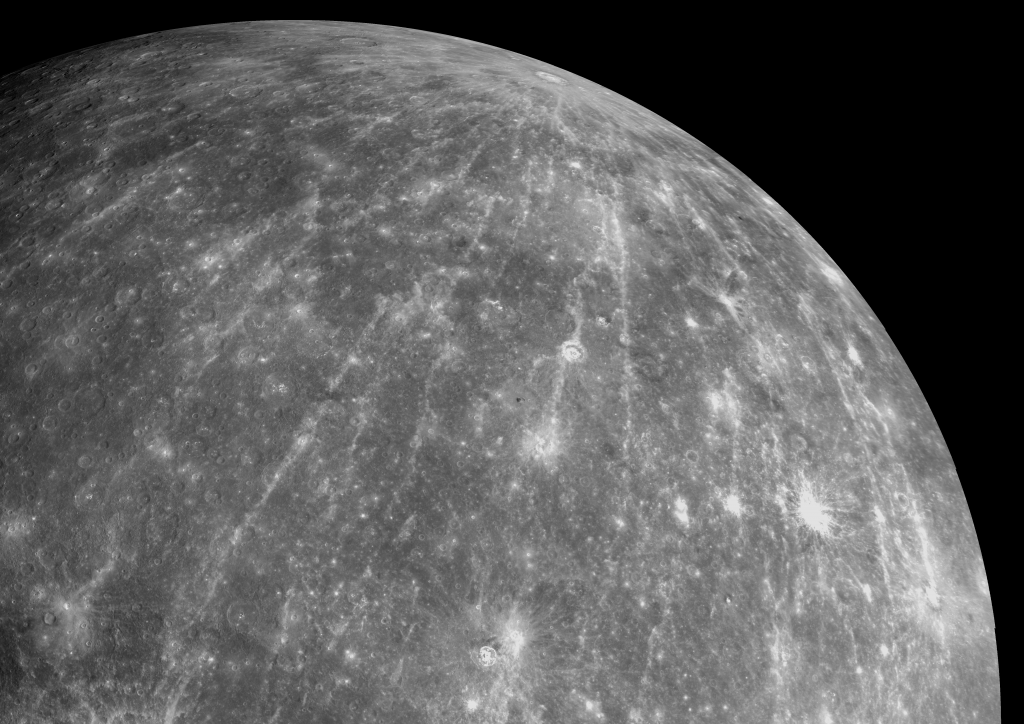
Impact crater Hokusai, located on Mercury at a latitude of 58°N, showing impressive system of rays
Where Did Mercury’s Ice Come From?
There are distinctive features of the ice deposits on Mercury, which have given the team clues as to their relative age. ‘The images showed very sharp boundaries between the shadowed ice deposits and neighbouring sunlit rock, providing strong evidence that the ice on Mercury must be fairly young to have such well-defined boundaries,’ explains Dr Ernst.
Other celestial bodies, such as comets and asteroids, frequently impact Mercury’s surface. When these impacts occur, they mix up the surface materials, causing the edges of features to appear irregular. As the ice deposit boundary edges have not yet been mixed up, it is likely that the ice was deposited either recently (within geological timescales) or that the ice and associated blanket of organic compounds are constantly renewed as part of an ongoing planetary process, such as outgassing or micro-meteorite bombardment.
The researchers have noted that not every permanently shadowed region at Mercury’s north and south poles has ice. This uneven distribution of ice, observed on both hemispheres, may also give clues as to how the ice came to be on Mercury. ‘Our continuing analysis of Mercury’s ice deposits suggests that Mercury’s ice is fairly young and perhaps delivered by a large comet or asteroid impacting Mercury within the last tens of millions of years or so,’ says Dr Chabot. Since the postulated organic compounds covering Mercury’s ice are similar to those found on comets, this further supports the idea that the ice was delivered by a large and relatively recent impact on Mercury.
In a recent study led by Dr Ernst, the team was able to identify the geologically young Hokusai crater as the most likely impact crater produced from a celestial body capable of delivering the ice. By studying the shape and characteristics of the crater and its ejecta, the team was able to estimate the mass of water that may have been delivered to Mercury by the impact. They calculated that a large projectile of up to 31 kilometres in diameter, travelling at over 100,000 kilometres per hour, could deliver a large amount of water that matches the estimated volume of water locked in the ice at Mercury’s poles.
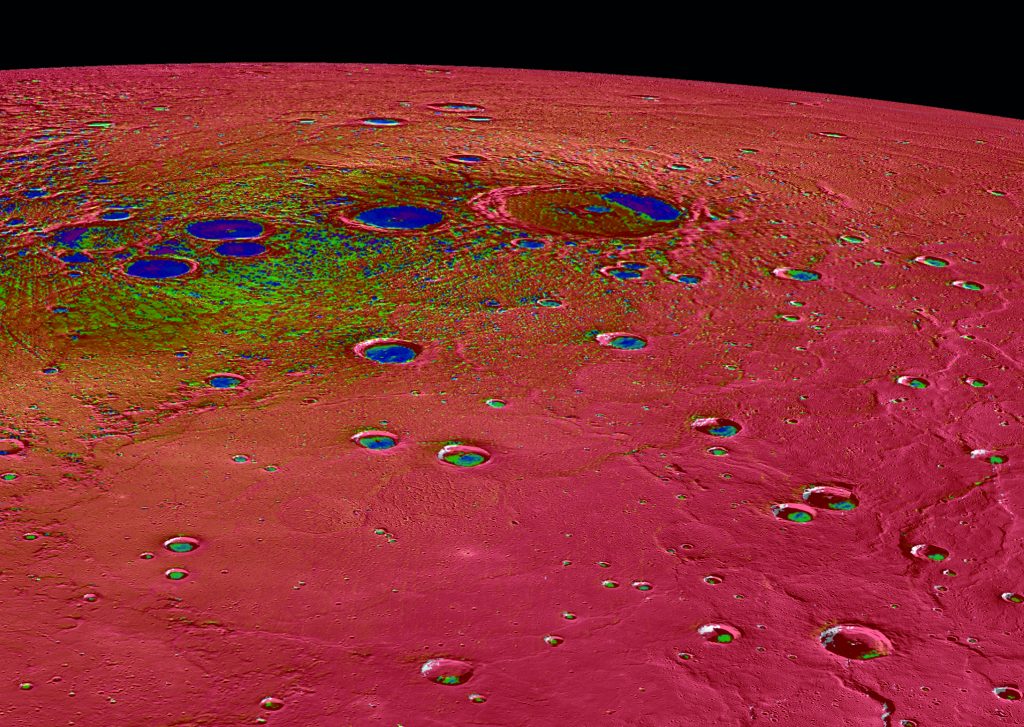
Mercury’s north polar region, coloured by the maximum biannual surface temperature, which ranges from more than 400 K (red) to 50 K (purple)
What’s Next?
The research completed by Dr Chabot, Dr Ernst, Deutsch and their colleagues into the origins of water ice on Mercury is an important piece in the puzzle of understanding where the water in our solar system came from. During the early evolution of the rocky inner planets, it was too hot for water to condense on Earth. The fact that Mercury’s ice may have been successfully delivered by a comet or asteroid impact suggests that the water on Earth may also have this distant origin. NASA has found exoplanets (planets beyond our solar system) in the early stages of formation that are undergoing a similar bombardment by primitive asteroids and comets that could be delivering water to their surfaces, in the same way that it was likely delivered to Earth.
While the mission for the MESSENGER spacecraft may be over, the research team’s mapping of Mercury’s poles will help to guide the next mission to Mercury. The European Space Agency and the Japan Aerospace Exploration Agency together are working on the BepiColombo spacecraft. Building on the discoveries of the MESSENGER mission, BepiColombo will orbit Mercury in 2025 for at least one Earth year, providing scientists with even more data about one of the solar system’s most mysterious planets.
Reference
https://doi.org/10.33548/SCIENTIA386
Meet the researchers
Dr Nancy Chabot
Johns Hopkins University Applied Physics Laboratory
Laurel, MD
USA
Dr Nancy Chabot is a planetary scientist at the Johns Hopkins University Applied Physics Laboratory. After gaining her PhD from the University of Arizona, she became involved with the NASA MESSENGER mission as the Instrument Scientist for MDIS, as well as chairing the Geology Discipline Group. Since 2016, Dr Chabot has continued to research the rocky planetary bodies in our solar system as Coordination Lead for NASA’s DART mission and as Deputy PI for the MEGANE instrument on the JAXA MMX mission. Dr Chabot is a fellow of the Meteoritical Society and also has an asteroid named after her: Asteroid 6899 Nancychabot.
CONTACT
W: https://secwww.jhuapl.edu/SpaceScience/staff/ProfileView/105
Dr Carolyn Ernst
Johns Hopkins University Applied Physics Laboratory
Laurel, MD
USA
Dr Ernst is a planetary scientist at the Johns Hopkins University Applied Physics Laboratory. She earned her PhD from Brown University, and her research investigates the processes and effects of impact cratering and the surface evolution of planetary bodies. She worked on the MESSENGER mission as Deputy Instrument Scientist of the Mercury Laser Altimeter, and is currently working on NASA’s Europa Clipper and DART missions. She is also a Participating Scientist on the Japan Aerospace Exploration Agency Hayabusa2 mission. Dr Ernst also has an asteroid named after her: Asteroid 11008 Ernst.
CONTACT
W: https://secwww.jhuapl.edu/SpaceScience/staff/ProfileView/57
Ariel Deutsch, PhD Candidate
Planetary Geoscience Group
Brown University
Providence, RI
USA
Ariel Deutsch is a PhD candidate at Brown University and a NASA Graduate Fellow at NASA Goddard Space Flight Center. Between 2013 and 2015 she worked with Dr Chabot and Dr Ernst in the MESSENGER Science Operations Center as a NASA/Applied Physics Laboratory Intern. At Brown, she is continuing to research Mercury’s polar deposits as one component of her doctoral thesis. She is particularly interested in the evolution of planetary bodies and in using remote sensing and geophysical techniques to understand their geological history.
W: https://www.arieldeutsch.com/
KEY COLLABORATORS
Olivier Barnouin, Johns Hopkins University Applied Physics Laboratory
David Blewett, Johns Hopkins University Applied Physics Laboratory
Brett Denevi, Johns Hopkins University Applied Physics Laboratory
John Harmon, Arecibo Observatory
James Head, Brown University
Erwan Mazarico, NASA Goddard Space Flight Center
Scott Murchie, Johns Hopkins University Applied Physics Laboratory
Hari Nair, Johns Hopkins University Applied Physics Laboratory
Gregory Neumann, NASA Goddard Space Flight Center
David Paige, University of California, Los Angeles
Evangela Shread, Johns Hopkins University Applied Physics Laboratory
Sean Solomon, Lamont-Doherty Earth Observatory, Columbia University
FUNDING
NASA
Creative Commons Licence
(CC BY 4.0)
This work is licensed under a Creative Commons Attribution 4.0 International License.
What does this mean?
Share: You can copy and redistribute the material in any medium or format
Adapt: You can change, and build upon the material for any purpose, even commercially.
Credit: You must give appropriate credit, provide a link to the license, and indicate if changes were made.
More articles you may like
How Food Environments Shape Our Eating Habits
How we eat dramatically impacts our health, yet millions of Americans live in ‘food deserts’ – areas with limited access to fresh, nutritious food. Recent research reveals that solving this crisis requires looking beyond just physical access to food to understand how our entire community environment shapes our dietary choices. Through a series of pioneering studies, Dr Terrence Thomas and colleagues at North Carolina A&T State University have been investigating how different aspects of our food environment influence what we put on our plates. Their findings suggest that creating lasting change requires reimagining how communities engage with food at every level.
Probing Electron Dynamics in the Ultrafast Regime
In the atoms that make up the matter around us, negatively charged particles called electrons have properties such as spin and orbital angular momentum. Researchers at Martin Luther University Halle-Wittenberg have developed a theoretical framework which allows them to simulate the dynamics of the spin and orbital angular momentum of electrons in materials when probed with an ultrafast laser pulse. Using this framework, they are able to simulate different materials and improve our understanding of dynamics on an atomic scale.
Seeing Beneath the Surface: Exploring Deltaic Reservoirs with Augmented Reality
In the Aínsa Basin of the Spanish Pyrenees, the Mondot-1 well was drilled, cored, and fully logged to capture a detailed record of a long-buried ancient river delta system. Dr. John D. Marshall, Dr. Jürgen Grötsch, and Dr. Michael C. Pöppelreiter with co-workers at Shell International used this core to trace how sediments once flowed across the landscape, and were deposited under shifting tectonic conditions. The team employed augmented reality and interactive virtual displays; these innovative tools offer new ways to explore subsurface depositional systems, and are particularly useful in locations where physical access to the core is difficult, or no longer possible.
Dr Jim Wu | Ziresovir Offers New Hope for Treating Respiratory Syncytial Virus Infections
Respiratory syncytial virus (RSV) causes respiratory tract infections in children and adults. While for many patients the outcomes of infection are mild, for others, infection can prove fatal, and there is a lack of effective treatments. Dr Jim Wu from the Shanghai Ark Biopharmaceutical Company in China carries out his vital research to develop new, safe, and effective treatments to tackle this killer.