Dr Philip Myers – A Family Affair: Exploring Early Star Formation
We know much about fully-formed stars, such as our Sun, but the very earliest processes of star formation are still a mysterious area of astrophysical research. The original idea that a single new-born star (or ‘protostar’) forms within a single molecular cloud core has been dispelled by the discovery of new-born pairs, triplets, or even larger groups of protostars in cores. Dr Philip Myers of the Center for Astrophysics | Harvard and Smithsonian has been observing and interpreting protostar formation for many years using a range of sophisticated telescopes and theoretical models.
Star Birth and Evolution
On a clear night, we can stand on the Earth’s surface and gaze into a night sky full of bright stars. In our galaxy alone, there are around 250 billion stars, which is just a mere fraction of the number of stars in the universe. Stars have their own particular lifecycle that varies in length depending on their mass. For example, our Sun, a relatively low-mass star, is already around 4.6 billion years old – about halfway through its lifecycle.
Stars are the powerhouses of our galaxy, spreading heavier elements such as carbon and oxygen into the planetary systems that form around them, and providing the light and heat required to support life. A star emits light due to the ongoing fusion reaction in its centre, where hydrogen is converted into helium. However, a star’s life begins long before it starts to shine.
Clouds of gas and dust, known as molecular clouds, are found throughout our galaxy and beyond. It is within these molecular clouds that protostellar objects, or protostars (the earliest stage of a star) are formed. ‘Stars and their planet-forming circumstellar disks form in “dense cores”, or regions of increased density in an interstellar molecular cloud,’ describes astrophysicist Dr Philip Myers of the Smithsonian Astrophysical Observatory, who studies the complex processes behind the early formation of stars.
Gravitational attraction causes this gas and dust to begin to draw together. As the material continues to collapse, it rotates, causing the densest gas to flatten into a disk-like shape. The material at the centre begins to heat up, forming a protostar surrounded by a disk of gas and dust that may eventually form orbiting planets.
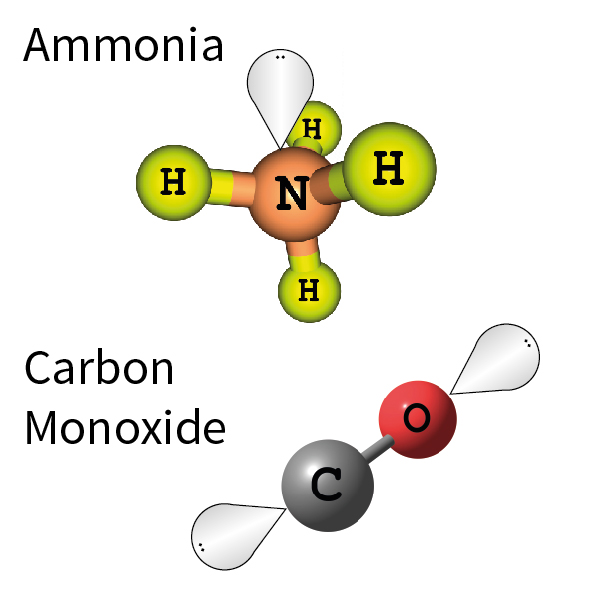
Key molecules for studies of star-forming gas.
Observing Protostars
Although nuclear fusion has not yet kick-started in a protostar, it still emits visible light, generated due to heating as the protostar contracts. However, this light is absorbed by the surrounding molecular cloud, which reradiates it as lower-energy radiation at infrared and submillimeter wavelengths. This means that we cannot see protostellar objects using normal optical telescopes.
Protostars were first studied in depth during the 1980s using new observation techniques that could measure this low-energy radiation. Specific molecules such as carbon monoxide and ammonia within the molecular clouds emit characteristic frequencies of radiation, allowing scientists to figure out exactly which molecules are present in clouds millions of kilometres away. The properties of this radiation also provide information about the density, temperature, and internal motions of the star-forming gas.
Using telescopes that can create images from this low-energy radiation, Dr Myers and his colleagues made observations of dense cores within dark molecular clouds, in their hunt for clues about early star formation. By studying the locations, masses, and stability of cores in the Taurus-Auriga dark cloud complex, they were able to predict that most, if not all of these cores would eventually form stars. This was one of the first times scientists had identified protostar-forming conditions within a molecular cloud.
In 1986, Dr Myers and his team were able to complete a survey of dense cores in nearby star-forming regions, using the Infrared Astronomical Satellite (IRAS). This was the first survey of its kind in the hunt to identify a protostar, as no previous search had the sensitivity to detect such cold, faint objects embedded in molecular clouds.
The team found that over one-third of molecular cloud cores in known stellar nurseries contained an infrared source that was a newly-formed star. They determined that the sources were embedded deep in the molecular cloud surrounded by cold shells of material and still in the very earliest accretion stages.
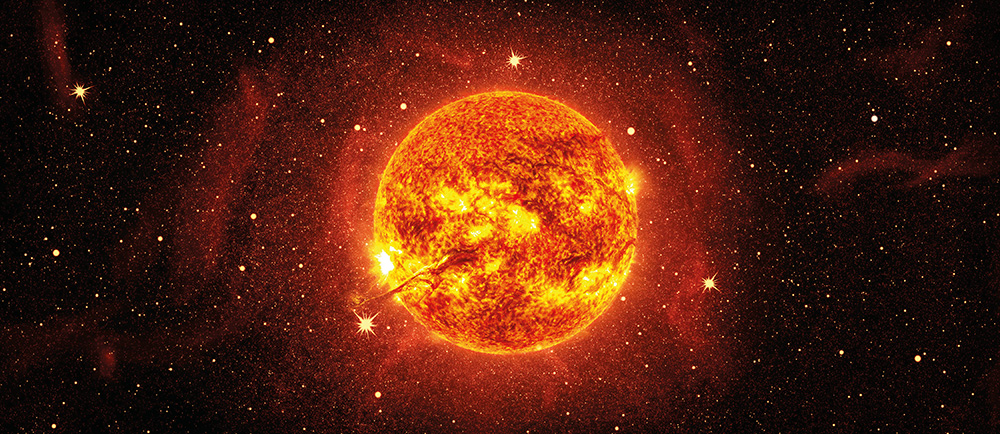
The sun, our nearest star, is more massive and much older than a typical protostar.
Brothers and Sisters
For many years, researchers relied on simple models where a spherical dense core undergoes gravitational collapse to form a single protostar at its centre. However, during the late 1990s, new observations led to some new ideas about protostar formation. Scientists studying dark molecular clouds known as Bok globules found clear evidence of multiple neighbouring protostars.
When scientists first imaged these Bok globules using infrared telescopes, they identified single protostars by their infrared emission and by their outflowing streams of gas, detected in spectral lines of the carbon monoxide (CO) molecule. However, more detailed observations soon showed that some of these protostars have near neighbours in the same dense core. ‘These discoveries opened the door to studies of protostar multiplicity, with recent surveys of star-forming regions revealing many systems of carbon monoxide outflow from binary or triple protostars,’ says Dr Myers.
Spitzer and Herschel
More recently, Dr Myers and his colleagues have focussed on dense cores that contain small groups of young stars. As telescopes have improved in sensitivity and image resolution, the team has been able to identify areas containing young star clusters. However, the early formation conditions of cores harbouring such protostar groups were still poorly understood.
In a recent 2019 study, Dr Myers and the team reported key features of protostars using infrared observations by NASA’s Spitzer Space Telescope. They surveyed the Gould Belt region, which is home to well-studied molecular clouds that contain the majority of currently forming stars closest to our Sun. From the survey data, the team identified 32 protostellar groups, five of which are extremely young, since they exclusively contain protostellar objects and no older objects. This study revealed new properties of these young groups, including their number, diameter, and surface density.
They then combined this information with a survey of the same areas completed by the Herschel Space Observatory. Run by the European Space Agency, this Observatory contained the largest mirror ever built for a space telescope mission. The telescope collected infrared radiation at longer wavelengths than did the Spitzer Space Telescope, allowing it to observe the gas and dust in extremely cold regions with greater sensitivity than did the Spitzer Space Telescope.
Dr Myers and his colleagues used these data to study the relationship between the protostellar objects and the density of the gas formed around them. The team determined that the groups showing both high protostellar surface density and high gas density are undergoing vigorous star formation. This identifies them as excellent targets for future studies investigating the formation processes of protostars.
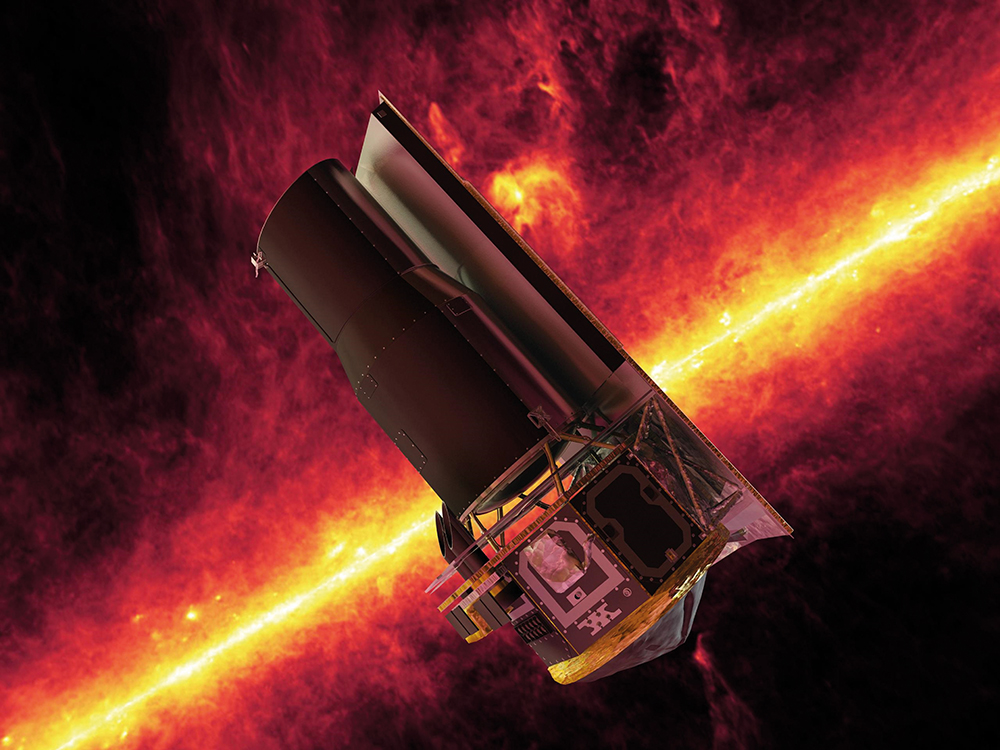
NASA’s Spitzer Space Telescope. CREDIT: NASA/JPL-Caltech
Fragmentation
The discovery that multiple protostars form together casts doubt on the traditional view that gravitational collapse of a spherical core leads to the creation of a single star. Instead, the well-known process of fragmentation appears increasingly important. During this process, molecular clouds break down into ever-smaller fragments, which then collapse to form protostars.
‘We now see more evidence of fragmentation – gravitational, magnetic, and turbulent processes in a dense region which create multiple centres of gravity rather than just one,’ says Dr Myers. He and his colleagues used new observations of the Perseus molecular cloud to study the process of fragmentation. They used a combination of previous observations from surveys completed by telescopes including the Spitzer Space Telescope along with new data taken from the MASSES project (Mass Assembly of Stellar Systems and Their Evolution with the Sub Millimetre Array).
The team identified a hierarchical structure of nested fragments within the cloud that covered five different size scales. The largest is the cloud itself, whose diameter is more than one million times the distance between the Earth and Sun. Clouds contain smaller clumps, which in turn house filaments. These filaments are much smaller but are still about three trillion kilometres in length. Inside filaments, there are much smaller fragments of molecular cloud, known as cores and envelopes. It is inside these envelopes that protostellar disks and stars are formed.
The team’s study, published in 2018, was the first of its kind to look in detail at the fragmentation structures within a single molecular cloud, paving the way for future research into cloud structure and the distribution of protostar masses.
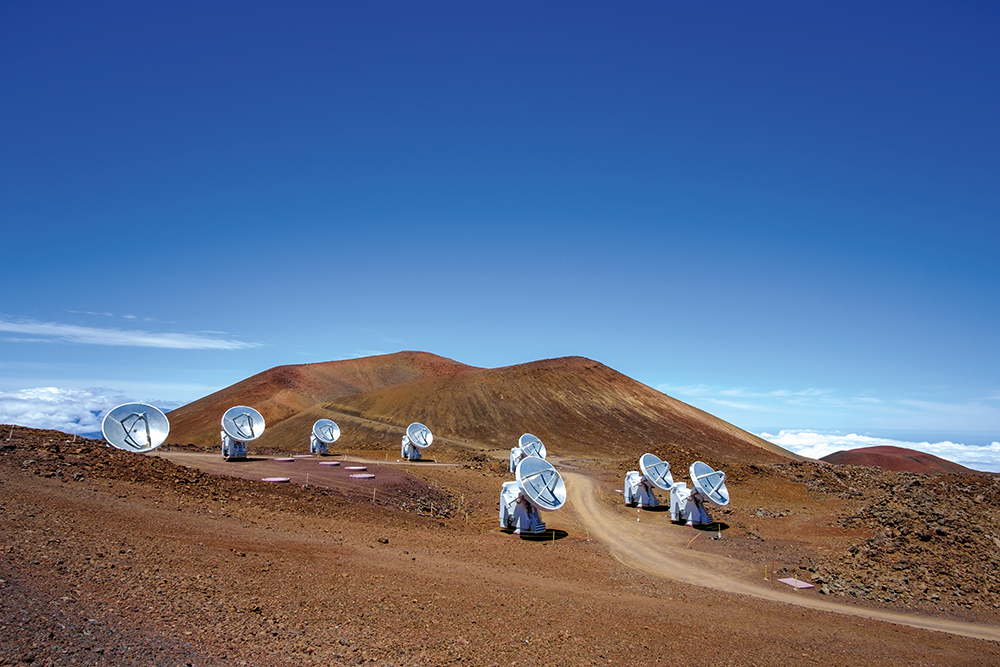
The Submillimeter Array atop Mauna Kea
Advancing the Field
To help fellow researchers in illuminating the mysteries of star formation, Dr Myers and his team have released the MASSES survey data as a publicly available dataset. The survey has imaged the 74 confirmed protostars within the Perseus molecular cloud, and it offers an enormous amount of information on the dust and gas around each protostar, including its structure and multiplicity of condensations, its chemical composition, its CO outflows, and the energy in its internal motions. Databases such as these are vital for collaborative research, and aid scientists across the globe as they shine a light into one of the most mysterious processes in our universe.
Reference
https://doi.org/10.33548/SCIENTIA423
Meet the researcher
Senior Astrophysicist Philip Myers
Center for Astrophysics | Harvard and Smithsonian
Cambridge, MA
USA
After completing a PhD in physics at the Massachusetts Institute of Technology, Dr Philip Myers joined the physics faculty there, before moving to Harvard University and the Smithsonian Astrophysical Observatory (SAO). His successful research career has led to over 200 publications, and he has been the recipient of many research awards. Dr Myers’ particular research focus is on stars and the processes driving their formation, combining observations with theoretical studies. He has also held Visiting Professor positions at numerous international universities, including the University of Leeds in the UK, the Lorentz Centre at Leiden University in the Netherlands, and the University of California, Berkeley. During his career, he has supported and supervised many early career scientists, who have themselves gone on to successful research careers in astrophysics.
CONTACT
KEY COLLABORATORS
Sayantan Auddy, Academia Sinica Institute of Astronomy and Astrophysics, Taiwan
Shantanu Basu, University of Western Ontario, Canada
Priscilla Benson, Wellesley College
Charles Beichman, NASA Jet Propulsion Laboratory
Tyler Bourke, Square Kilometer Array, Manchester, UK
Michael Dunham, State University of New York, Fredonia
Robert Gutermuth, University of Massachusetts, Amherst
Helen Kirk, Herzberg Institute, Victoria, Canada
Juan Li, Shanghai Astronomical Observatory, China
Riwaj Pokhrel, University of Toledo
Sarah Sadavoy, Queen’s University, Canada
Ian Stephens, Center for Astrophysics | Harvard and Smithsonian
FUNDING
SAO
NASA
FURTHER READING
J Li, PC Myers, H Kirk, R Gutermuth, MM Dunham, R Pokhrel, Catalog of High Protostellar Surface Density Regions in Nearby Embedded Clusters, The Astrophysical Journal 2019, 871, 163
IW Stephens, TL Bourke, MM Dunham, PC Myers, and 12 co-authors, Mass Assembly of Stellar Systems and their Evolution with the SMA – Full Data Release, to appear in The Astrophysical Journal Supplement Series, 2019.
R Pokhrel, PC Myers, MM Dunham, IW Stephens, SI Sadavoy, Q Zhang, TL Bourke, JJ Tobin, KI Lee, RA Gutermuth, SSR Offner, Hierarchical fragmentation in the Perseus Molecular Cloud: From the cloud scale to protostellar objects, The Astrophysical Journal, 2018, 853, 5.
H Kirk, PC Myers, Young Stellar Groups and Their Most Massive Stars, The Astrophysical Journal, 2011, 727, 64.
TL Bourke IRAS 11590-6452 in BHR 71: A Binary Protostellar System? The Astrophysical Journal, 2001, 554, L91.
PC Myers, PJ Benson, Dense Cores in Dark Clouds. II. NH3 Observations and Star Formation, The Astrophysical Journal, 1983, 266, 309.
Creative Commons Licence
(CC BY 4.0)
This work is licensed under a Creative Commons Attribution 4.0 International License.
What does this mean?
Share: You can copy and redistribute the material in any medium or format
Adapt: You can change, and build upon the material for any purpose, even commercially.
Credit: You must give appropriate credit, provide a link to the license, and indicate if changes were made.
More articles you may like
Grandmothers: Innovation Through Tradition
Grandmother Project – Change through Culture (GMP) is an organisation dedicated to documenting the role of grandmothers and demonstrating the effectiveness of grandmother-inclusive strategies in improving the health and well-being of women, children, and adolescents. GMP’s groundbreaking work challenges conventional wisdom to transform community-based interventions in Africa and beyond, harnessing a powerful but often overlooked resource: the wisdom and influence of grandmothers.
Dr Robert Larkin | Cultivating Change to Improve Soil Health and Increase Potato Yield
Environmental quality and food production are facing the pressing challenges of climate change and global population growth. Dr Robert Larkin from the United States Department of Agriculture-Agricultural Research Service (USDA-ARS) and a team of plant scientists developed and tested a range of crop management systems to help overcome these compounding challenges. Their work is improving soil health and increasing the yield of potato crops, contributing to the future food security of nations.
Professor Giorgio Buttazzo | Artificial Intelligence and a Crossroads for Humanity
Where do we stand with artificial intelligence? Might machines take over our jobs? Can machines become conscious? Might we be harmed by robots? What is the future of humanity? Professor Giorgio Buttazzo of Scuola Superiore Sant’Anna is an expert in artificial intelligence and neural networks. In a recent publication, he provides considered insights into some of the most pressing questions surrounding artificial intelligence and humanity.
Dr Ralf Adam | New Technologies Shaping the Future of Oral Hygiene
Understanding the efficiency of various toothbrush technologies is essential for achieving optimal oral health. Dr Ralf Adam, who leads a dedicated team at Procter & Gamble in Germany, is keen to investigate the complexities of these technologies. His team have provided new insights into the best toothbrush types for plaque removal and the maintenance of gum health. By highlighting the importance of informed oral care decisions and ongoing investigations, this vital research works towards ensuring everyone can achieve a brighter, healthier smile.