Dr Susan Voglmaier – Driving Forward Training and Research in Psychiatry
In recent years, dramatic advances have been made in brain science and molecular genetics. However, there is currently a shortage of psychiatrists with the scientific training necessary to take this knowledge and apply it in the clinic. Psychiatrist and neuroscience researcher, Dr Susan Voglmaier of the University of California, San Francisco, runs a research training program that supports the next generation of research scientists in the field of psychiatry. Dr Voglmaier believes that by training doctors in scientific techniques and methods, we may come to better understand mental illness and provide more effective treatments for psychiatric diseases in the future.
Training the Next Generation of Psychiatrists
The Psychiatry Research Resident Training Program at the University of California, San Francisco (UCSF) is a specialised program that aims to increase the number of qualified research psychiatrists. The program also focuses on fostering the integration of biology and psychology in advancing the treatment of neuropsychiatric disease. Dr Susan Voglmaier directs the program, where she oversees the research and clinical training of psychiatrists. Participants of the program are MDs, many of whom already have PhDs, who undertake a comprehensive training program that provides them with neuroscience or clinical research training and career mentorship in classroom, laboratory, and clinical settings. Partially funded by the National Institute of Mental Health, the program includes neuroscience workshops, leadership experiences, and individualised training to allow psychiatrist-scientists to establish and maintain the running of scientific research projects alongside their clinical training.
Neurotransmitters: Deciphering Their Role in Mental Illness
In addition to leading the Research Resident Training program, Dr Voglmaier runs an active research lab that focuses on the study of specialised proteins that are found inside of nerve cells (neurons) in the brain, called vesicular neurotransmitter transporters. Neurotransmitters are chemical signals that are transmitted rapidly (on the order of milliseconds) from one neuron to another, coordinating learning, memory, and action. Glutamate, an amino acid, is the most abundant neurotransmitter in the human brain and mediates neuronal excitation. Inside the cell, vesicular glutamate transporters (VGLUTs) package glutamate into specialised sacs or compartments called synaptic vesicles. When neurons receive electrical signals from other neurons, synaptic vesicles fuse with the cell membrane and release glutamate from the cell. When glutamate is detected by the receptors on a neighbouring neuron, this results in signal transmission. After neurotransmitter release, synaptic vesicles are broken down, reformed, and refilled with neurotransmitter for a new round of release.
There are three types of vesicular glutamate transporter, known as VGLUT 1, 2 and 3, with VGLUT1 and VGLUT2 being the most abundant. These two glutamate transporters are expressed in different areas of the brain as well as at different levels and times during the life of an organism. During development, VGLUT2 levels are highest in the womb, while VGLUT1 levels increase during brain maturation and are higher in adults compared to younger individuals. In general, VGLUT2 is expressed in glutamatergic pathways that transmit sensory information from the outside world, while VGLUT1 pathways transmit information from memory about meaning.
A precise balance of VGLUT1 and VGLUT2 containing synaptic terminals is needed to integrate information and coordinate output behaviour. For example, when a person sees a round, red object this information is transmitted via VGLUT2 pathways and is compared to information in the brain carried by VGLUT1 pathways, to identify whether the object is a ball or an apple, and whether s/he should throw it or eat it (Figure 1).
The formation of new synaptic vesicles and their loading with glutamate need to keep up with the rapid signaling demands of the cell. If this doesn’t happen, insufficient glutamate will be available for signal propagation. In the central nervous system, imbalance of glutamate signaling at the synapse is suspected to be involved in schizophrenia, major depressive disorder, and bipolar disorder. VGLUT1 and VGLUT2 each have sophisticated signals in their structure that control the take-up, transport, and release of glutamate from the synaptic vesicle as well as their sorting to reforming synaptic vesicles after they have fused with the cell membrane.
At UCSF, Dr Voglmaier’s research team have unravelled a number of the molecular mechanisms that allow glutamatergic synaptic vesicle proteins to recycle from the cell membrane at the synapse and allow glutamate to refill vesicles.
In recent studies, her team found that although the basic process of glutamate transport across the synaptic vesicle membrane by both forms of glutamate transporters is identical, the recycling of the synaptic vesicle from the cell membrane at the synapse of the neuron proceeds in very distinct ways depending on whether VGLUT1 or VGLUT2 is involved. The researchers showed that the speed of take-up and recycling of the transporters differs between VGLUT1 and VGLUT2 even in the same cell type, demonstrating that the proteins themselves control the rate of their recycling. This is an important and new finding, as previous studies had assumed that all synaptic vesicle proteins recycled equivalently. However, the scientists found that VGLUT1 exhibits faster rates of recycling than VGLUT2.
These differences in the speed of vesicle recycling likely shape the rate, amount, and pattern of how much glutamate is released at the synapse and that will subsequently be available for cell signaling. Synapses that contain VGLUT1 exhibit a lower initial probability of release of glutamate while synapses with higher levels of VGLUT2 exhibit a higher probability of release. By exploiting the acidic environment inside the synaptic vesicle and using fluorescent genetically-encoded tools to track VGLUTs under the microscope, Dr Voglmaier’s team were able to determine the makeup and function of the sorting signals and discovered additional proteins that interact with the two types of VGLUTs and mediate the differences between them.
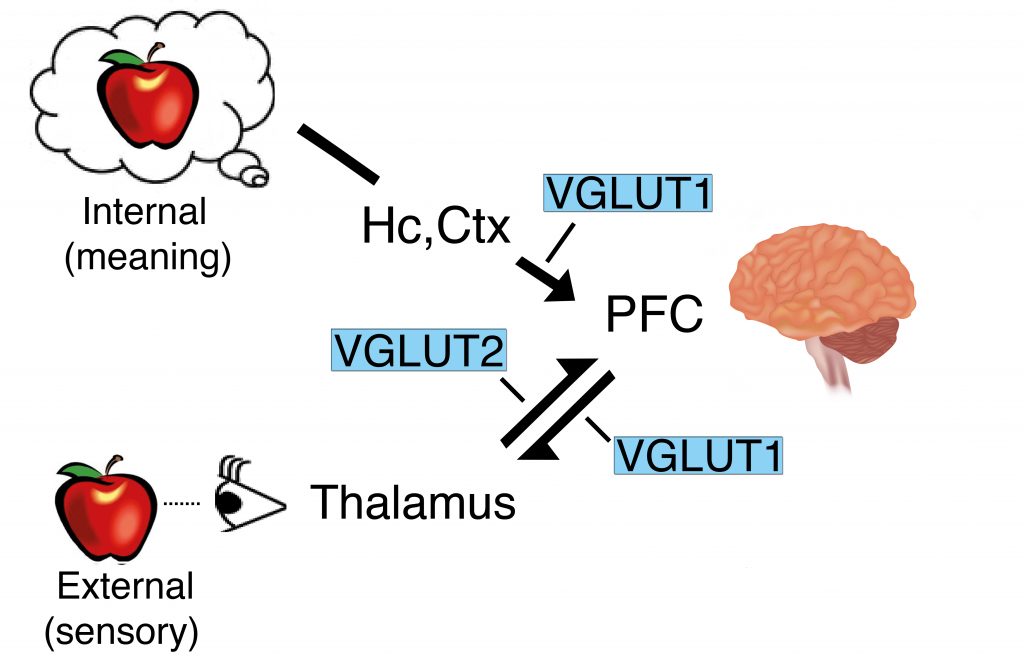
Figure 1. The VGLUT1 and VGLUT2 pathways. Credit Susan Voglmaier.
VGLUTS and the Synaptic Vesicle Cycle
Structurally, VGLUT1 and VGLUT2 are identical with the exception of a few molecular differences at the head and tail ends of the proteins. The tail end of VGLUT1 is slightly different from that of VGLUT2, containing protein interaction domains not present in VGLUT2, and that appear to contribute to the faster recycling of VGLUT1. However, little is known about the mechanisms underlying VGLUT2 recycling. This motivated Dr Voglmaier and her team to examine how VGLUT2 recycles across synaptic membranes using the protein pHluorin.
The protein pHluorin, a pH-sensitive form of green fluorescent protein, is used in neuroscience to study neurotransmitter release. Dr Voglmaier and her team designed specialised forms of pHluorin for their studies. These consist of pHluorin fused to the side of the VGLUT facing the inside of the synaptic vesicle. The pH inside transmitter vesicles is acidic, and the VGLUT-pHluorin is non-fluorescent under these conditions. When vesicles are released, VGLUT-pHluorin is exposed to the extracellular space, which has a neutral pH, and the presynaptic terminal becomes brightly fluorescent. Following the re-internalization and reformation of the synaptic vesicles, the vesicles become re-acidified and the cycle can start again (Figure 2). The fusion of a green fluorescent molecule to VGLUT1 and VGLUT2 allowed the researchers to track their activities during neuronal signal transmission and determine how they influence the interactions of synaptic vesicle proteins, the reformation of synaptic vesicles, and the release of glutamate.
Findings from these studies confirmed that VGLUT2 recycles differently than VGLUT1. These results indicated that it is protein identity, not synaptic vesicle membrane or neuronal cell type, that controls sorting the rate and method of VGLUT uptake and release, and that VGLUT2 relies on distinct recycling mechanisms from VGLUT1. In addition, the team was able to identify interactions with other proteins that were crucial for VGLUT1 trafficking at the synaptic membrane.
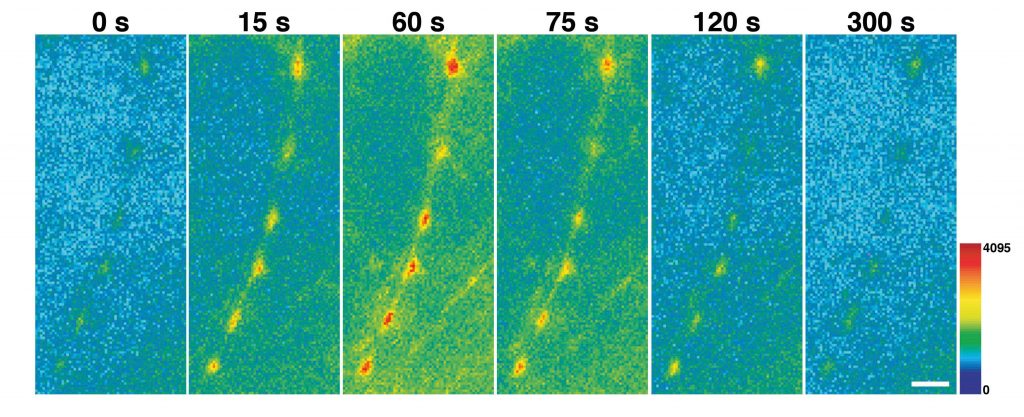
Figure 2. VGLUT-pHluorin exposed to the extracellular space. Credit Susan Volgmaier.
Synthesised Medicine
When the biochemical mechanisms of a particular disease are elucidated, specialised drugs can be designed to target the condition at a microscopic level. These synthesised drugs can be made in the lab via a series of chemical reactions using molecular building blocks to create larger molecules with the desired makeup and confirmation. Examples include molecules with the potential to block a particular molecular mechanism from occurring by binding to a target, at the site of the reaction or elsewhere. These drugs can be tailored to be delivered at specific potencies to the tissue where they are needed, leaving surrounding cells unaffected.
The rate of modern drug synthesis and development methods continue to gain momentum, along with novel delivery and targeting systems, such as nanotechnology. The first step in drug creation and development is the study and understanding of the process of disease. Dr Voglmaier hopes that her work will lead to a sufficient understanding of the molecular pathways that could be exploited to invent new drugs to increase or decrease the recycling of glutamate and other important neurotransmitters at the synapse level.
The Future of Medicine and Mental Health
The research in Dr Voglmaier’s lab continues to provide excellent training for scientists in the field of neurobiology. In addition, through the UCSF Department of Psychiatry’s Research Resident Training Program she provides training and support to a new generation of psychiatric clinician-scientists, who work at all levels of analysis, from molecular and cellular biology, to human participant research, to health systems. Dr Voglmaier thus contributes to the development of mental health specialists with the ability to understand and integrate their psychiatric clinical practice with laboratory, clinical, and health services research. We can hope that other institutions will follow the UCSF blueprint created by Dr Voglmaier. As scientists work towards a better understanding of the brain biochemistry and molecular mechanisms of neuropsychiatric disease, it is hoped that the ability to synthesise specialised medicines for major mental illnesses, which have so far evaded effective treatment, will become the norm.
Reference
https://doi.org/10.33548/SCIENTIA426
Meet the researcher
Professor Susan Voglmaier
University of California, San Francisco
Weill Institute for Neurosciences
Kavli Institute for Fundamental Neuroscience
Department of Psychiatry
San Francisco, CA
USA
Professor Susan Voglmaier completed her MD and PhD in Neuroscience at the John Hopkins School of Medicine in 1997. She then completed her medical residency in psychiatry at the University of California, San Francisco (UCSF), where she also worked as a clinical and research fellow in both Psychiatry and Neurology. Dr Voglmaier is currently an attending psychiatrist in the Adult Psychiatric Clinics at UCSF where she specialises in psychotic, anxiety, and mood disorders and performs resident supervision and medical student teaching. In addition, she is an Associate Professor in the Department of Psychiatry at UCSF, where she studies the regulation of synaptic vesicle recycling and neurotransmitter release, methods to image these processes, and animal and cellular disease models. She has also been a Faculty Member in the Neuroscience Program at UCSF since 2007 and is the Co-Director of the Psychiatry Research Resident Training Program.
CONTACT
http://neurograd.ucsf.edu/people/susan-voglmaier-md-phd
http://psych.ucsf.edu/rtp/research
T: @UCSFRRTP
FUNDING
National Institutes of Mental Health
BBRF/NARSAD
Brain Research Foundation
International Mental Health Research Organization (IMHRO)
March of Dimes
UCSF Program for Breakthrough Biomedical Research
Whitehall Foundation
FURTHER READING
H Li, MS Santos, CK Park, et al, VGLUT2 trafficking is differentially regulated by adaptor proteins AP-1 and AP-3, Frontiers in Cellular Neuroscience, 2017, 11, 324.
AD Besterman, JK Williams, VI Reus, et al, The role of regional conferences in research resident career development: The California Psychiatry Research Resident Retreat, Academic Psychiatry, 2017, 41, 272–277.
MS Santos, H Li, SM Foss, SM Voglmaier, Protein interactions of the vesicular glutamate transporter VGLUT1, PLoS One, 2014, 9, e109824.
SM Foss, H Li, MS Santos, et al, Multiple dileucine-like motifs direct VGLUT1 trafficking, Journal of Neuroscience, 2013, 33, 10647–60.
MS Santos, CK Park, SM Foss, et al, Sorting of the vesicular GABA transporter to functional vesicle pools by an atypical dileucine-like motif, Journal of Neuroscience, 2013, 33, 10634–46.
H Li, SM Foss, YL Dobryy, et al, Concurrent imaging of synaptic vesicle recycling and calcium dynamics, Frontiers in Molecular Neuroscience, 2011, 4, 34.
Z Hua, S Leal-Ortiz, SM Foss, et al, v-SNARE composition distinguishes synaptic vesicle pools, Neuron, 2011, 71, 474–87.
Creative Commons Licence
(CC BY 4.0)
This work is licensed under a Creative Commons Attribution 4.0 International License.
What does this mean?
Share: You can copy and redistribute the material in any medium or format
Adapt: You can change, and build upon the material for any purpose, even commercially.
Credit: You must give appropriate credit, provide a link to the license, and indicate if changes were made.
More articles you may like
Dr Timothy Beers | Mapping the Galaxy’s Stellar Populations Using Large Photometric and Astrometric Surveys
Astronomers often use spectroscopic (electromagnetic radiation) data and astrometric (motion and positional) data to develop working models describing our Galaxy. Dr Timothy Beers from the University of Notre Dame and his collaborators in Korea and China combined large photometric (visible light) surveys and astrometric data to create multidimensional maps of a large part of the Galaxy. By highlighting significant inhomogeneities in stellar-chemical compositions, motions, and spatial distributions, Dr Beers and his colleagues provide valuable insights into how we can advance our understanding of the formation and evolution of our Galaxy.
Dr Anushia Inthiran | Distance Learning: Impacts for Offshore Students Amid COVID-19
The COVID-19 pandemic significantly disrupted global education and necessitated a shift to online learning. Due to ongoing border closures, even after the pandemic eased, offshore students were prevented from attending their university in person long after their local peers, impacting their learning ability and future perspectives. Dr Anushia Inthiran from the University of Canterbury conducted a survey among a group of offshore students to understand the consequences of distance learning on their education.
Dr Sébastien Weber | PyMoDAQ: Navigating the Future of Data Acquisition
In an era where data is paramount, Dr Sébastien Weber and his team at CNRS, the French National Centre for Scientific Research, are changing the landscape for scientists and engineers with PyMoDAQ, an open-source data acquisition software. Their revolutionary tool stands out for its accessibility, versatility, and the thriving community it fosters.
Professor Gary Yohe | Navigating Climate Change: The Impactful Contributions of Gary Yohe
Professor Gary Yohe is a distinguished environmental economist whose work has been pivotal in shaping our understanding of climate change impacts, adaptation strategies, and policy frameworks. His interdisciplinary approach combines economics with environmental science, offering nuanced insights into global warming and its multifaceted impacts on natural and human systems. Professor Yohe equips us with the knowledge and strategies needed to navigate the complex and pressing challenges posed by climate change.