Professor Michel Moisan | Providing Stable and Power-Efficient Plasma Using Microwaves
The ability to generate stable and reproducible plasma is central to many aspects of research and technology. Through his research, Professor Michel Moisan and his team at Université de Montréal (UdeM) explored the capabilities of various devices they patented that produce plasma columns simply and efficiently, using radiofrequency or microwaves. Applications of these devices range from the sterilisation of medical equipment, to purifying noble gases such as xenon for ion-thrusters that ensure the repositioning of communication satellites.
Generating Plasmas
Devices that can generate plasma have been continuously developed over the last few decades, particularly in welding, lasers and material processing. To generate plasma, tools developed at Université de Montréal (UdeM) take a neutral gas in a cylindrical tube, and subject it to electromagnetic ‘surface waves’ – typically either radiofrequency or microwaves – that run along the tube.
The electromagnetic surface waves initially accelerate a few electrons that are randomly present in the gas, which then go on to strip the outer electrons of atoms in a source gas, creating an ‘avalanche’ process, which culminates in a stationary fluid of electrons and ions – a plasma.
In many applications such as lighting, the generated plasma is contained inside the tube. If the process of sustaining plasma is based on electromagnetic surface waves, the tube must be transparent to radiofrequency or microwaves. It must be made from a so-called dielectric material, such as fused silica, or a ceramic that is more resistant to high temperatures and chemicals. For other applications, such as the sterilisation of medical devices, the tube must be open-ended, allowing the flowing plasma to emerge in the processing chamber.
For plasma generation to work effectively, it is crucial for power to be transferred from the radiofrequency or microwave generator to the plasma as efficiently as possible, while also ensuring that stable gas discharges can be sustained for extensive periods of time. In addition, this must be done across a broad range of operating conditions – including varying compositions and pressures in the gas. Plasma etching, for example, which is employed in fabricating the tiny circuits on computer chips, requires abrupt changes to the composition of the input gas during the manufacturing process. To operate effectively, plasma-generating devices must withstand these types of changes, without running off or substantially increasing their power requirements.
In 1991, Professor Michel Moisan and his colleague Professor Zenon Zakrzewski first reviewed the operation principles and design of surface-wave plasma sources. Their paper has since given rise to 84 mentions in patent applications, highlighting the ubiquity of this technology in various fields.
Introducing the Surfaguide
In 2006, Professor Moisan, student Thomas Fleisch, and their colleagues at UdeM fully characterised the surfaguide field applicator, and turned it into a simple, compact and efficient design that could overcome many previous challenges. The surfaguide design (Fig. 1) starts with a rectangular waveguide, where an aperture is situated through a section of the waveguide wide wall. A movable short-circuit wall, or ‘plunger’, allows the device to be matched with the microwave power generator.
The generated plasma column extends to both sides of the surfaguide aperture. With these features in place, the device can transfer power from microwaves to plasma at close to 100% efficiency, even across the broad range of sudden changes in operating conditions encountered during the various sequences of plasma-driven processes.
‘It is possible to find surfaguide design and dimensions such that it currently runs in factories at a 6 kilowatt power level under abrupt changes in operating conditions, such as gas composition and pressure, without having to retune the plunger, ensuring constant impedance matching with the power generator,’ says Professor Moisan.
Understanding Plasma Discharge Properties
It had previously been shown that, for given operating conditions, the discharge properties were independent of the specific surface wave generator used. However, it was generally believed that the properties of the propagating surface wave were directly affected by the plasma columns they generated.
This was wrong. In one of his studies, Professor Moisan revisited this assumption by observing experimentally that the electron density decreased linearly along the plasma column, and produced such a plasma along its whole length to the very end. Upon examining this under a variety of operating conditions (gas nature and pressure, wave frequency, discharge tube inner radius), it became clear that the surface wave was sustaining the gas discharge without experiencing any interaction from the medium it created.
‘This interpretation can be considered a paradigm shift, since most theorists come out with an axial distribution of electrons that curves down toward the end of the plasma column, contradicting observations,’ explains Professor Moisan.
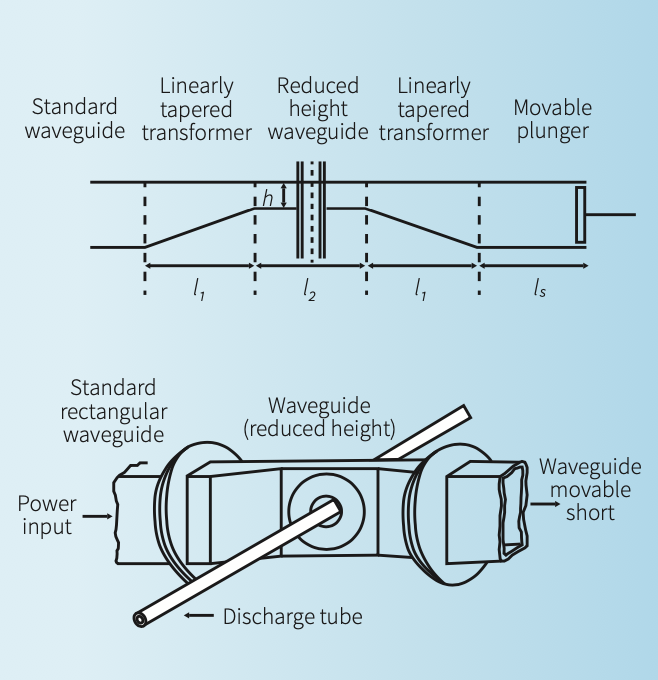
Figure 1: Schematic illustration of the surfaguide
Microwaves vs Radiofrequency
Leading on from this study, Professor Moisan and his team next examined the advantages of using microwave produced plasmas, as opposed to those generated using radiofrequencies. Alongside collaborators abroad, Ganachev and Nowakowska, the researchers revealed several, unexpected and unique properties pertaining to microwave sustained discharges. ‘These result from their distinct features,’ explains Professor Moisan. ‘For one, the microwaves only transfer energy to electrons, since the heavier positively-charged ions can’t respond to rapid field oscillations occurring above 100 megahertz. Secondly, the plasmas created through microwave discharges have stationary and smaller dimensions of their “plasma sheath” layers – a region of positive ions at the vessel wall in contrast to neutrality within the whole plasma.’
Choosing between microwave and radiofrequency discharges depends on the application desired. For example, radiofrequency discharges are essential in computer chip manufacturing due to the ion bombardment that is generated in the sheath. Etching the chip substrate in this way using radiofrequency-generated plasma provides the required patterning to ensure the proper chip architecture.
On the other hand, applications including the remediation of greenhouse gases and the production of new materials are better off with microwave discharges, mainly due to the relatively higher electron density of such plasmas.
Through their analysis, Professor Moisan and his colleagues introduced a new model called the ‘Concept of power per electron’, which unveils several useful key features of microwave-generated plasmas. In contrast to radiofrequency plasmas, these features include the fact that almost no electron energy needs to be expended to sustain the plasma sheath, thus leading to higher electron density. Additionally, the power absorbed per electron in these discharges has been shown to adjust to compensate for the power losses – the dominant feature in this power balance.
The team also showed that absorbing power across a confined volume within the larger plasma volume increases the electric field intensity in the smaller volume, thereby offering the possibility to increase atomic excitation and ionisation.
With all of these important insights into how power is transferred through microwave-sustained discharges, researchers are now better placed to explore their applicability across a range of important scenarios.
Sterilising Medical Equipment
Among the many possible uses of the plasma columns produced by surface waves is the sterilisation of medical equipment. ‘Sterilisation implies that at the most, only a single microorganism is left among initially one million,’ explains Professor Moisan. With existing techniques, this often involves treating equipment with intense heat or high-energy gamma radiation, which risks damaging the apparatus. These risks can be overcome by treating equipment with ethylene oxide, but this gas is explosive, toxic, and carcinogenic. These shortcomings create strong incentives to find an alternative.
In one study, Professor Moisan and his team used the surfatron, a lower-frequency microwave field applicator than the surfaguide. They used this device to apply microwaves to an optimised mixture of nitrogen and oxygen, to generate a plasma column that emitted an intense afterglow of ultraviolet light. ‘The afterglow designated the region along the column where no electric field from the surface wave is left,’ says Professor Moisan.
Ultraviolet light with wavelengths of between 180 and 350 nanometres induces lesions in the DNA of microorganisms, ultimately leading to their inactivation. This light inactivates any bacteria or viruses it interacts with, as well as infectious proteins, such as the prion that causes Creutzfeldt-Jakob Disease.
The advantage of the surfatron sterilisation technique over all other plasma sterilisation systems is that it relies on the far flowing afterglow (Fig. 2). ‘This means that at a long enough distance from the microwave field applicator, there are no longer any electrons in the flowing gas,’ says Professor Moisan. ‘Otherwise, as we demonstrated, electrons charge up on microorganisms, which are then released by an electrostatic force acting on the substrate so rapidly that they do not become inactivated, eventually contaminating the process chamber and flowing out into the outside world.’
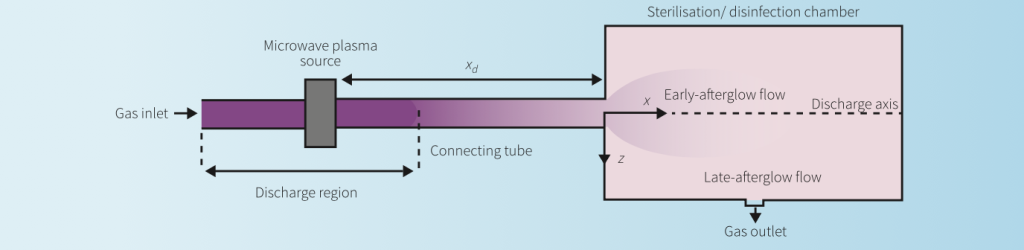
Figure 2: Representation of the discharge outflow of long-lived species as they enter the sterilisation/disinfection chamber
after travelling from the microwave-plasma source.
Eliminating Greenhouse Gases and Purifying Rare Gases
In another study, Professor Moisan’s team examined how plasmas could be used to remove fluorinated greenhouse gases such as CF4 and SF6, which can remain in the atmosphere for over 50,000 years before degrading. These two gases are used in the plasma reactors required to fabricate computer chips.
In their experiment, to avoid damage to the pumping system, the team ‘drowned’ these fluorinated gases in a nitrogen stream requiring plasma effluents carried in rapid nitrogen flows. ‘The process, which takes place at atmospheric pressure with the addition of a few percent of oxygen, is ecologically clean and electrically very efficient when compared to burners previously used,’ explains Professor Moisan. ‘The surfaguide system is employed industrially in abating fab-reactor greenhouse gases as well as purifying noble gases’.
Future Opportunities
The technology of microwave plasma discharges, which has not been exploited as much as that of radiofrequency discharges, seems to offer more green and efficient prospects in plasma-driven processes. ‘It provides high purity rare gases, a new approach to plasma sterilisation of medical devices and clean microelectronic fabrication facilities,’ concludes Professor Moisan. ‘In addition, new materials such as graphene and carbon tubes, for example, can be obtained at a higher rate with microwave plasmas when exploited according to the Concept of power per electron, a noteworthy model applicable to all kinds of microwave-produced discharges.’
Among the various existing field applicators, Professor Moisan states that the surfaguide is the simplest one to build and operate. ‘The surfaguide has in fact become a standard laboratory tool as shown by the fact that its patented origin is no longer mentioned in publications,’ he continues. ‘Another potential application under development in various countries is the use of microwave plasma antennas for communication; monopole antennas can be deployed as a plasma column and transmit a message in less than a few microseconds.’
SHARE
DOWNLOAD E-BOOK
REFERENCE
https://doi.org/10.33548/SCIENTIA869
MEET THE RESEARCHER
Professor Michel Moisan
Groupe de physique des plasmas
Université de Montréal
Montréal, Québec
Canada
Professor Michel Moisan achieved a Doctorat d’État (Doctor of Science) in Plasma Physics at the Université Paris-Sud (now Université Paris-Saclay) in 1971. After graduation, he was invited as Guest of the Academy of Sciences of the USSR. In mid-October 1972, he joined the Groupe de physique des plasmas at the Université de Montréal, where he currently serves as Professor Emeritus in Physics. In 2008, he also co-founded, with J. Pelletier, the International Laboratory for Plasma Technologies and Applications (LITAP), which is now an International Research Group. Professor Moisan’s research interests include the use of high-frequency electromagnetic fields (radiofrequencies and microwaves) to sustain gaseous discharges, as well as the science and applications of cold gaseous plasmas up to atmospheric pressure. He has been conferred the title of Chevalier dans l’Ordre des Palmes académiques de la République Française in 2017, received the 2017 Innovation Award of the European Physical Society (Plasma Physics division) and the France-Québec Prix Adrien-Pouliot for scientific collaboration with France in 2005.
CONTACT:
E: michel.moisan@UMontreal.CA
KEY COLLABORATORS
Professor Emeritus Joseph Hubert, Chemistry Department, Université de Montréal
Professor Antonia Shivarova (deceased), Faculty of Physics, St. Kliment Ohridski university, Sofia, Bulgaria
Science Doctor Jacques Pelletier, CNRS Research Director, Université Joseph Fourier, Grenoble, France
Science Doctor André Ricard, CNRS Research Director, Université Paul Sabatier, Toulouse, France
Professor Zenon Zakrzewski (deceased), Institute of Fluid Flow Machinery, Polish Academy of Sciences, Gdansk, Poland
Dr Jean-Christophe Rostaing (deceased), Centre de Recherche Claude-Delorme (CRCD), Air Liquide, Les-Loges-en-Josas, France
Dr Helena Nowakowska, Institute of Fluid Flow Machinery, Polish Academy of Sciences, Gdansk, Poland
FUNDING
Conseil de Recherche en Sciences Naturelles et Génie (CRSNG) du Canada (NSERC). Individual research grant: ‘Caractérisation expérimentale et modélisation des décharges entretenues par des champs HF (RF et micro-ondes), conception de nouveaux types de décharges HF et applications des décharges HF à certaines études ou procédés’ (Experimental characterisation and modelling of discharges maintained by HF fields (RF and microwaves), design of new types of HF discharges and applications of HF discharges to certain studies or processes), uninterrupted grant from 1977 to 2021. Additionally, NSERC Strategic Grants, e.g. diamond deposition with Surface Wave Discharges.
Fonds Québécois de la Recherche sur la Nature et les Technologies (FQRNT), Québec government team grants.
Industrial grants (major ones): IBM, Central Lab., Yorktown Heights, NY USA) 1984-1988, 250 k$; Imperial Chemical Industries (ICI), Manchester, UK. 1985-1988, 160 k$;
Air Liquide, Paris, France. 1997-2005, 3.47 M$.
REPUBLISH OUR ARTICLES
We encourage all formats of sharing and republishing of our articles. Whether you want to host on your website, publication or blog, we welcome this. Find out more
Creative Commons Licence (CC BY 4.0)
This work is licensed under a Creative Commons Attribution 4.0 International License.
What does this mean?
Share: You can copy and redistribute the material in any medium or format
Adapt: You can change, and build upon the material for any purpose, even commercially.
Credit: You must give appropriate credit, provide a link to the license, and indicate if changes were made.
SUBSCRIBE NOW
Follow Us
MORE ARTICLES YOU MAY LIKE
Dr Olalla Castro-Alvaredo | Measuring Entanglement: Symmetry-Resolved Entropy
Dr Olalla Castro-Alvaredo of the City University of London (UK) and her collaborators are advancing our understanding of an important phenomenon of quantum mechanical systems known as entanglement and, especially, its mathematical measures. Symmetry-resolved entanglement entropy is one such measure. Their study focuses on special quantum states which are excited with respect to a ground state. The research shows how the entanglement amongst quantum particles can be measured and assesses the contribution to the entanglement of quasiparticle excitations, particularly in the presence of additional symmetries.
Dr Tsun-Kong Sham – Dr Jiatang Chen – Dr Zou Finfrock – Dr Zhiqiang Wang | X-Rays Shine Light on Fuel Cell Catalysts
Understanding the electronic behaviour of fuel cell catalysts can be difficult using standard experimental techniques, although this knowledge is critical to their fine-tuning and optimisation. Dr Jiatang Chen at the University of Western Ontario works with colleagues to use the cutting-edge valence-to-core X-ray emission spectroscopy method to determine the precise electronic effects of altering the amounts of platinum and nickel in platinum-nickel catalysts used in fuel cells. Their research demonstrates the potential application of this technique to analysing battery materials, catalysts, and even cancer drug molecules.
Dr Marcus Noack and Dr Mark Risser | Advancing Gaussian Processes: The Noack-Risser Method
Dr Marcus Noack and Dr Mark Risser, researchers at Lawrence Berkeley National Laboratory, have recently proposed a significant advancement in the area of machine learning and data science that promises significant computational improvements: the enhancement of exact Gaussian Processes for large datasets, significantly improving data analysis capabilities for samples even beyond 5 million data points.
Dr Gregory Duane | Predicting Climate Change with Supermodels
Our universe is comprised of fascinatingly complex systems. Systems such as the Earth’s climate can, at first glance, seem far too complex and chaotic to predict accurately. Dr Gregory Duane and his team at the University of Colorado have been developing complex computational models that can learn from past data, providing us with intriguing insights and more accurate predictions about the future.