Professor Wilfred D. Stein – Using Quantitative Tools to Learn More About Genes
Applying mathematics to biology can help reveal the elusive details of life. Professor Wilfred D. Stein and his colleagues from the Hebrew University of Jerusalem and institutes worldwide have used quantitative tools to tackle topics ranging from cancer treatment to drug-resistant malaria, and lately, the evolution of the human genome. His extensive and broad work has supported scientists in many different areas of biomedicine and will leave a lasting impact on life science.
Biomathematics: A Far-reaching Science
The work of Professor Wilfred D. Stein, Professor Emeritus at the Hebrew University of Jerusalem, has covered ground far and wide, from the genetics of cancer to drug-resistant malaria. A common thread in his work has been the use of mathematics to find objective answers to key questions. ‘What I do is to apply quantitative methods to problems of biology,’ explains Professor Stein. The mathematics used by Professor Stein tends to focus on tumour growth, protein interactions and gene evolution, aspects of biology which are very amenable to mathematical modelling.
One of Professor Stein’s recent projects on gene ageing emphasises the utility of quantitative tools in studying biology. Estimating the age of genes can help further our understanding of their role but would be nigh on impossible without the quantitative tools which have been developed by biomathematicians.
A Look Back in Time
A gene’s age is determined by how far back in time it can be first identified in an ancestor of the organism in question. This can be achieved using the extensive and widely available genomic databases. Taking advantage of these resources, in 2018 with his colleague Professor Litman, Professor Stein published a list of the ages of all the protein-coding genes and many of the non-protein coding genes in the human genome.
Professor Stein wanted to use this gene ageing technique to learn more about cancer-associated genes and when they arose in the evolutionary tree. Genes are a tricky thing to study, and only recently have scientists developed ways to do so. Genomic phylostratigraphy is a statistical method which looks at the way genes evolve by comparing them across species and ancestors throughout time. Genes, like us, are the descendants of ancestors, not exact copies, but similar enough to see their relationship.
When studying evolution, scientists can draw up a tree of ancestors for an organism, much like a family tree. Humans, at the very top of the tree, are of the order primates, which evolved from the placental mammals, which evolved from terrestrial vertebrates, and so on, all the way back to the last universal common ancestor. This common ancestor, known as LUCA, was likely a single-celled organism living in the deep ocean which would eventually give rise to every organism we know today.
Organisms on the tree can then be sorted into clades, a group which shares a recent common ancestor. Humans and chimpanzees are in the same clade because we share an ancestor, just like how you and your cousins are in the same family when you share a grandfather or a grandmother.
‘Clades are nested, one in another,’ Professor Stein notes. New clades form as evolution proceeds, new characteristics appear and populations diverge and evolve independently. Like sets of nesting dolls, the outermost clade, or the largest doll, contains all living organisms. Each clade contains all the descendants of one common ancestor. In the family tree example, your extended family clade is comprised of you and all the descendants of your grandfather, while your immediate family clade only includes you and your siblings, the descendants of your father or mother.
‘I have been able to estimate the ages of the genes that have been added, over the ages, to the evolving human genome.’
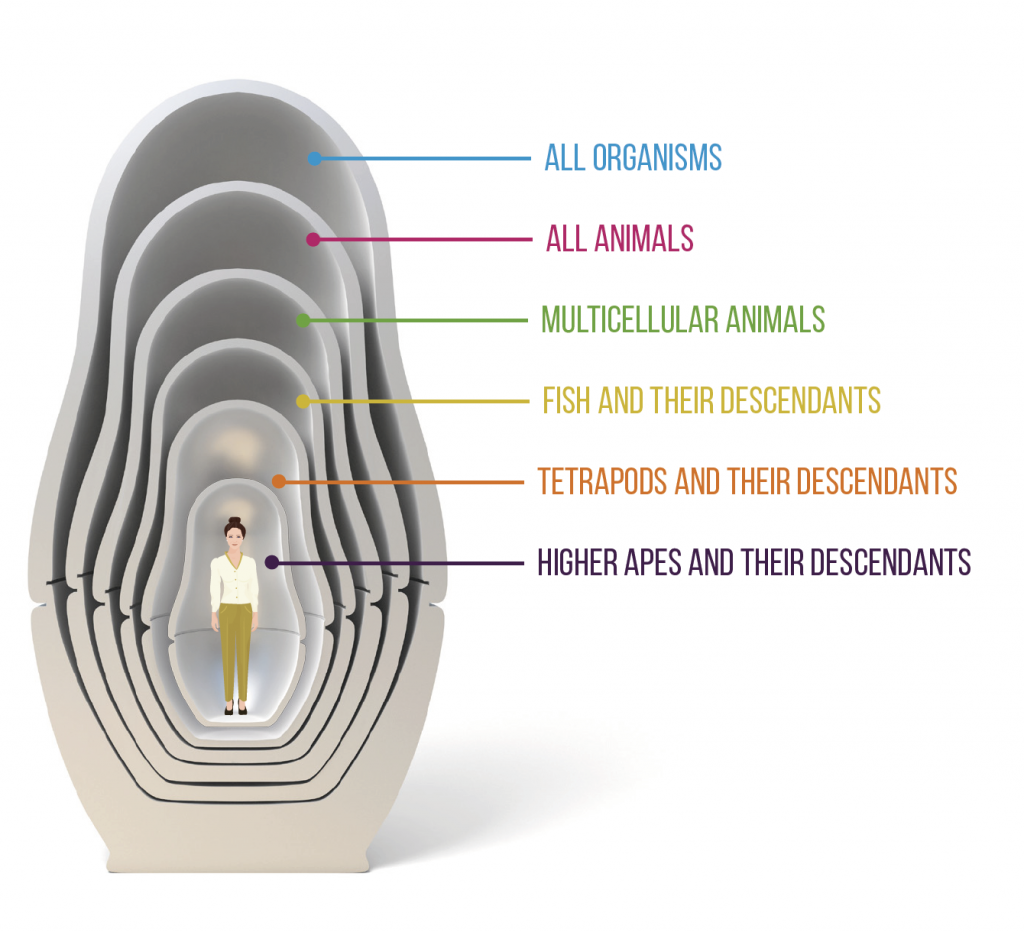
New clades form as evolution proceeds. The outermost clade contains all living organisms.
The organisms which have not ‘evolved out’ of their clade can be considered as being in a phylostratum level. ‘A phylostratum level is the set of organisms that first appeared in a newly-formed clade and remained in that clade, while some of their descendants evolved new characteristics to form the organisms of the next succeeding clade,’ explains Professor Stein. ‘The organisms that remained behind, between one clade and the next, form the new phylostratum level’.
This concept of phylostratification is a valuable tool for scientists who attribute ages to genes. Genes are passed on to descendants in vertical evolution. Over time they may change slightly, perhaps making their function more efficient, but still effectively doing the same job. Some changes, however, can allow the taking on of a new function, making this into a brand new gene. Alternatively, a quite new gene can emerge, an ‘orphan gene’, arising from a DNA sequence that did not code for anything. A new gene, and its descendants that retain the same function, are known as orthologs.
So, a gene can be given an age by identifying the phylostratum where its founder gene, the earliest ortholog, first appeared. These ‘origin’ orthologs are found by searching online databases of entire genomes of living organisms, using a genomics tool called BLAST. By inputting the sequence of your gene of interest, BLAST finds genes with similar sequences which are likely to be the gene’s ancestor. By identifying the earliest phylostratum that this ancestor is in, we can obtain an estimate of how old it is.
Identifying gene ages using orthologs enables us to develop our understanding of evolution and has helped us learn more about the relationships between organisms. However, while this technique is useful, many think it is too much of an inaccurate science, and disputes in the scientific community mean that there is no agreed list of orthologs.
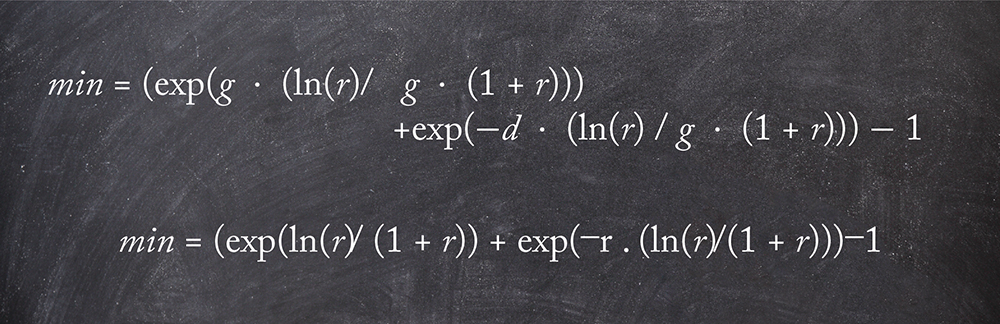
Equations illustrating the growth of cancers in response to chemotherapy.
Ancient Cancer Genes
‘By building on a consensus of the findings of the ortholog researchers, one can estimate the ages of the genes that have been added, over the ages, to the evolving human genome,’ elaborates Professor Stein, ‘and I have used this information to estimate ages of genes that, when mutated, drive cancer in the human body.’
The genetics of cancer has been of interest to researchers ever since cancer was first found to have a heritable element. Discovering when these genes may have arisen in evolutionary history may help us understand more about them.
Cancer-associated genes can be grouped into two classes with distinct and important roles in cell survival. Caretaker genes control things like the frequency of mutations which occur when cells replicate, while gatekeeper genes control cell growth. Mutations in these genes can result in a dysregulation of these important processes and cause excessive cell proliferation, or tumour growth. There are some thousand or more cancer-associated genes, many of which contribute just slightly to the possibility that someone will develop cancer.
With all the available tools at his disposal, Professor Stein and his co-workers began looking for the point at which cancer-associated genes arose in what would eventually become the human genome. They found that not only did cancer genes appear much earlier than would be expected, the genes associated with different types of cancers appeared at different points in time.
Carcinomas (cancers of the inner and outer linings of the body) and lymphomas (that arise from immune cells) are associated with genes regulating cell proliferation which appeared in the genome when organisms became multicellular. Many of the lymphoma specific genes appeared slightly later, at a point when the immune system developed in fish. Indeed, Professor Stein suggests that the earlier appearance of carcinoma genes is due to their more general function in terms of regulating activity at a point when cell differentiation was emerging in the first multicellular organisms.
Professor Stein’s research adds to our current understanding of the way the human genome evolved from all our previous ancestors. With cancer, it may be the case that mutations cause a breakdown in communication between our originally unicellular genes and multicellular genes. Uncovering these links can help in combatting this disease.
Predictive Thinking
Professor Stein’s work didn’t start there, however. Before his interest in gene evolution became fully realised, he was applying algorithms to better understand tumour growth. He derived a set of equations to measure the rate at which a tumour regrows following chemotherapy and demonstrated how this rate better predicts the patient’s outcome. This is in contrast to current approaches, where doctors look at the extent to which a tumour is reduced by treatment. Professor Stein further elaborates, ‘We found that the best predictor of the subsequent fate of patients treated with chemotherapy was the rate of regrowth of the tumour.’
In many cases, if a tumour is not reducing significantly in response to chemotherapy, the treatment is deemed ineffective and may be stopped. Professor Stein suggests that by looking at the regrowth rate, we can continue treatment if the tumour is growing slower than it would using other drugs. This could help prolong the life of patients and may mean that more chemotherapy drugs in the pipeline have a chance at making it to the clinic.
These kinds of mathematical tools can help determine better clinical endpoints, the point at which treatment is considered effective, and can help us make more informed decisions about what to look for when assessing drug efficacy. ‘Only if there is a better drug out there, with a lower growth rate than the drug being used should the therapy be changed,’ says Professor Stein, ‘I think this is one of the most important of my findings over the years.’
Malaria and Beyond
Professor Stein’s research has taken him through a myriad of important topics. He has also dedicated time to the study of drug-resistant malaria and developed kinetic equations to show how the parasite’s transporter proteins remove antimalarials from their site of action. Knowing this, scientists can now begin researching novel drugs to combat what has become an anti-malarial resistance crisis.
Currently, Professor Stein is applying the gene ages approach to elucidate the evolution of hair cells that were an invention of the mammals. Thus, he very much remains an active contributor to research and continues to bring his unique quantitative perspective to problems of biology.
Reference
https://doi.org/10.33548/SCIENTIA607
Meet the researcher
Professor Wilfred D. Stein
Professor Emeritus
Department of Biological Chemistry
The Silberman Institute
Hebrew University of Jerusalem
Jerusalem
Israel
Professor Wilfred D. Stein received his MSc in Physiological Chemistry from the University of Witwatersrand in 1954 and his PhD from King’s College London in Biophysics in 1958. His postdoctoral work took him to the Universities of Cambridge and Michigan Ann Arbor before he took up the position of Assistant Professor at the University of Manchester. In 1968 he joined the faculty of the Hebrew University of Jerusalem where he worked until his retirement, teaching biochemistry, biophysics, and physiology. His research has focused on using quantitative tools to investigate problems of biology and has ranged from the kinetics of tumour regrowth to determining the ages of genes with phylogenetic techniques. Professor Stein is the author of more than 300 peer-reviewed publications and nine books.
CONTACT
E: wilfostein@gmail.com
KEY COLLABORATORS
Dr Susan Bates, National Cancer Institute, NIH, Bethesda, USA
Dr Tito Fojo, National Cancer Institute, NIH, Bethesda, USA
Professor Michael Lanzer, Parasitology Department, University of Heidelberg, Germany
Dr Cecilia Sanchez, Parasitology Department, University of Heidelberg, Germany
Professor Thomas Litman, Department of Immunology, University of Copenhagen, Denmark
Dr Moshe Hoshen, Information systems and Computation Support, Ministry of Health, Jerusalem, Israel
Professor Hagai Ginsburg, Silberman Institute of Life Sciences, Hebrew University, Jerusalem, Israel
Further Reading
WD Stein, The Ages of the Cancer-Associated Genes, Seminars in Oncology, 2019, 46, 10–18.
T Litman, WD Stein, Obtaining estimates for the ages of all the protein-coding genes and most of the ontology-identified noncoding genes of the human genome, assigned to 19 phylostrata, Seminars in Oncology, 2019, 46, 3–9.
WD Stein WD, T Litman, Channels, Carriers, and Pumps: An Introduction to Membrane Transport, 2nd Edition, Academic Press, 2015.
CP Sanchez, CH Liu, S Mayer, et al, A HECT Ubiquitin-Protein Ligase as a Novel Candidate Gene for Altered Quinine and Quinidine Responses in Plasmodium falciparum, PLOS Genetics, 2014, 10(5), e1004382.
WD Stein, H Huang, M Menefee, et al, Other Paradigms: Growth Rate Constants and Tumor Burden Determined Using Computed Tomography Data Correlate Strongly with the Overall Survival of Patients with Renal Cell Carcinoma, Cancer Journal, 2009, 15(5), 441–447.
WD Stein, WD Figg, W Dahut, et al, Tumor Growth Rates Derived from Data for Patients in a Clinical Trial Correlate Strongly with Patient Survival: A Novel Strategy for Evaluation of Clinical Trial Data, The Oncologist, 2008, 13, 1046–1054.
WD Stein, Thinking About Biology, Westview Press, 1993.
WD Stein, Transport and Diffusion Across Cell Membranes, Academic Press, 1986.
WD Stein, The Movement of Molecules Across Cell Membranes, Academic Press, 1967.
Want to republish our articles?
We encourage all formats of sharing and republishing of our articles. Whether you want to host on your website, publication or blog, we welcome this. Find out more
Creative Commons Licence
(CC BY 4.0)
This work is licensed under a Creative Commons Attribution 4.0 International License.
What does this mean?
Share: You can copy and redistribute the material in any medium or format
Adapt: You can change, and build upon the material for any purpose, even commercially.
Credit: You must give appropriate credit, provide a link to the license, and indicate if changes were made.
More articles you may like
Dr Timothy Beers | Mapping the Galaxy’s Stellar Populations Using Large Photometric and Astrometric Surveys
Astronomers often use spectroscopic (electromagnetic radiation) data and astrometric (motion and positional) data to develop working models describing our Galaxy. Dr Timothy Beers from the University of Notre Dame and his collaborators in Korea and China combined large photometric (visible light) surveys and astrometric data to create multidimensional maps of a large part of the Galaxy. By highlighting significant inhomogeneities in stellar-chemical compositions, motions, and spatial distributions, Dr Beers and his colleagues provide valuable insights into how we can advance our understanding of the formation and evolution of our Galaxy.
Dr Anushia Inthiran | Distance Learning: Impacts for Offshore Students Amid COVID-19
The COVID-19 pandemic significantly disrupted global education and necessitated a shift to online learning. Due to ongoing border closures, even after the pandemic eased, offshore students were prevented from attending their university in person long after their local peers, impacting their learning ability and future perspectives. Dr Anushia Inthiran from the University of Canterbury conducted a survey among a group of offshore students to understand the consequences of distance learning on their education.
Dr Sébastien Weber | PyMoDAQ: Navigating the Future of Data Acquisition
In an era where data is paramount, Dr Sébastien Weber and his team at CNRS, the French National Centre for Scientific Research, are changing the landscape for scientists and engineers with PyMoDAQ, an open-source data acquisition software. Their revolutionary tool stands out for its accessibility, versatility, and the thriving community it fosters.
Professor Gary Yohe | Navigating Climate Change: The Impactful Contributions of Gary Yohe
Professor Gary Yohe is a distinguished environmental economist whose work has been pivotal in shaping our understanding of climate change impacts, adaptation strategies, and policy frameworks. His interdisciplinary approach combines economics with environmental science, offering nuanced insights into global warming and its multifaceted impacts on natural and human systems. Professor Yohe equips us with the knowledge and strategies needed to navigate the complex and pressing challenges posed by climate change.