Professor Richard Klemke | Targeted Drug Delivery: From Science Fiction to Reality
Most human diseases are localised in terms of their location but currently, injected or orally administered drugs are evenly distributed all over the body and thus, act indiscriminately. The targeted delivery of medication to the exact site where it is needed is a common theme in science fiction but thanks to Professor Richard Klemke and his team at the University of California San Diego’s Moores Cancer Center, this fantasy may soon become a reality.
The Fiction
Most of us will be familiar with the recurring scenario in science fiction: the protagonist, struck down with some fatal disease or injury, is saved miraculously by an ingenious drone programmed to navigate through the blood vessels, arrive at the site of the problem, and release the necessary drug just in time. Our hero survives another day. But is there any element of truth in this popular storyline that holds such promise for the future of medicine?
The Reality
The reality is that our ability to deliver drugs to precisely where they are needed in the body is very limited. The main problem is that most diseases are localised. Cancer, for example, usually affects only one site. Arthritis, most infections, diseases of the nervous system, and, in fact, just about any disease you can think of, will involve either one site or a limited number of select locations. Yet, in the absence of a better option, drugs are administered systemically. Irrespective of whether taken orally, injected into the blood, into a muscle or under the skin, medication is absorbed, circulates in the blood, and is deposited indiscriminately, everywhere in the body.
This approach has two significant shortcomings. First, a very large proportion of the drug ends up in places where it is not needed. This is not only wasteful but can be outright damaging for the patient. Take cancer again as an example. In an ideal world, we would want all cancer-killing chemicals concentrated within the tumour to provide the best chance of a cure because when the drug goes everywhere, it also affects other tissues. Problematically, cancer-killing chemicals in the bone marrow can switch off the production of red blood cells, causing anaemia. Elsewhere, damage to cells lining the gut can cause nausea and weight loss. An unwanted effect in the skin is hair loss. Other vital organs, the kidneys, the heart muscle, nerves, and hormone-producing organs can all be affected by indiscriminate drug distribution, causing severe side effects.
For these reasons, physicians try to delicately balance the dose of medication to ensure that it is suitably effective while also, minimising unwanted side effects. Unfortunately, such compromises limit our ability to treat many serious diseases effectively. Although researchers have tried several methods to overcome these problems (for example, utilising nanotechnology and microscopic fat droplet-mediated drug delivery), the results have usually been poor.
Starting with Mesenchymal Stem Cells
Professor Richard Klemke at the University of California San Diego’s Moores Cancer Center is a world leader in this exciting field. He explains that the starting point for his work was a fairly simple observation regarding mesenchymal stem cells (MSCs) involved in tissue regeneration. For example, when we cut a finger, MSCs move to the site of the injury and repair the damage by turning into cells that are needed locally and releasing beneficial substances.
MSCs caught Professor Klemke’s attention because here, he noted, is a cell that can move, can find its way to an injury, and can release large amounts of chemicals on the spot. Was there a way to utilise these MSCs for the targeted delivery of specific drugs?
Theoretically, it all seemed possible although there were considerable challenges to be overcome. For a start, MSCs repair damage by turning into other cells that are lost during the initial injury and uncontrollably produce unwanted factors. This ability is not only unnecessary for the purposes of targeted drug delivery but is also potentially damaging. As such, the accumulation and long-term survival of such cells and their secreted products could alter structures or cause other harm.
The genetic programme necessary for these unwanted effects is coded in the nucleus of the MSCs. Professor Klemke saw that the solution to several problems associated with using nucleated cells as therapeutic delivery devices was simply to remove the dangerous DNA from the cell. By removing the entire nucleus through a process known as cell enucleation, he reasoned that the cell would be rendered safe with a lower potential for carcinogenicity. Cell enucleation would also block new gene transcription, preventing the induction of potentially unwanted products after the cell was introduced into the body. Finally, the enucleated cell would have a defined life span which would make drug delivery more controlled and predictable.
But key questions remained, including the issue of how long the cells would survive after enucleation, whether they would still perform the critical migration functions necessary for locating and physically moving into disease tissues, and whether they would produce and deliver a defined therapeutic at the site of disease as intended. But, if the enucleated cells could survive and perform as hoped, then possibly, they might act like delivery drones dedicated to bringing defined therapeutics to diseased tissues in a much safer, controlled, and predictable manner than previously possible.
Professor Klemke’s team developed the necessary technology to achieve this by optimising the conditions for gently removing the nucleus from the cell. The resulting structures could no longer be considered cells, as they couldn’t divide, survive for long, or develop independently. Due to their intended use, the team decided to call these nucleus-free, cargo-carrying cell-derived particles CargocytesTM. The removal of the nucleus meant that most of the regulatory concerns and risks associated with cell therapy would no longer apply, making it easier to gain regulatory approval for their use in the future.
Professor Klemke and the team undertook rigorous functional testing to determine the therapeutic utility of the enucleated cells using animal systems – and this worked pretty well. But excitingly, the researchers knew they could substantially improve this process even further by introducing a GPS-like system to guide cells to diseased tissues using gene and cell engineering technologies.
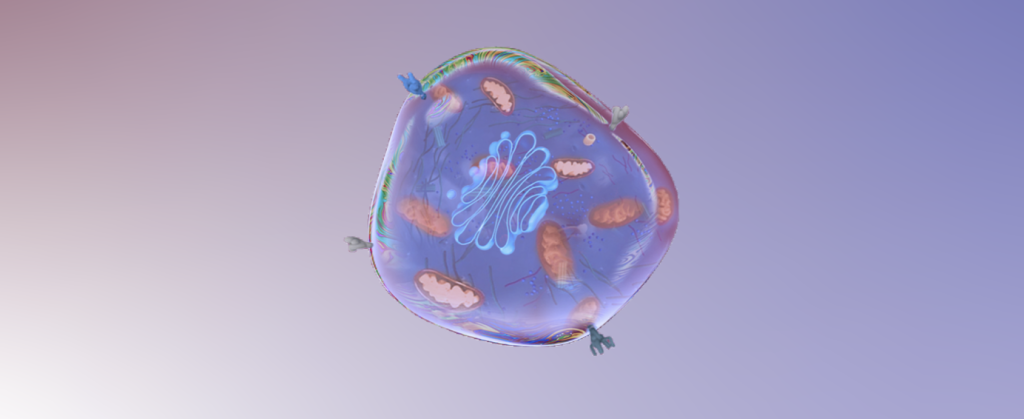
Illustration of a CargocyteTM. Reproduced with permission from Richard Klemke.
The Guidance System
While CargocytesTM have the necessary machinery to move, their use for drug delivery required a way to guide them to the location where they are needed. The biology behind this is complex. Imagine that there is an infection in your right earlobe, while CargocytesTM intended to treat this infection are swimming around in your blood, inside blood vessels. How would a CargocyteTM ‘know’ where to come out and deposit its content?
What helps is that there is a signal coming from the infected earlobe, alerting other cells to the problem. Biologists refer to these signals as chemokines. The first thing the team needed to do was to provide the CargocyteTM with the ability to detect these signals. Molecules with this sensory function are called chemokine receptors. Perhaps it is easiest to imagine these as antennae on the CargocyteTM surface, allowing it to detect the presence of a chemokine.
Once the CargocyteTM reaches the location where the chemokine signal is the strongest, there needs to be a mechanism that prevents it from being swept along with the blood flow. Rather, it needs to stop and then move out of the blood vessels passing near the diseased tissue. There are two types of molecules involved in this process: adhesion molecules and selectins. Adhesion molecules can be thought of as ‘hands’ holding onto selectins, microscopic ‘grab handles’. Adhesion molecules attaching themselves to selectins allow the CargocyteTM to stop and ‘climb’ in the required direction.
During initial experiments, Professor Klemke and his team were able to insert three different chemokine receptors – ‘antenna’ – onto the surface of CargocytesTM. They also showed that these structures were functional, in other words, that the modified CargocytesTM detected and responded to chemokine guidance signals, adhered to diseased blood vessels, and moved out of the vascular system into the diseased tissue
These experiments were first attempted in Petri dishes but encouraged by their early success, Professor Klemke and the team then conducted experiments in mice. Here, one ear of the animals was deliberately irritated, causing a mild inflammation. When unmodified CargocytesTM were injected into these animals, they did not leave the bloodstream. However, CargocytesTM modified with the right chemokine receptors and adhesion molecule rapidly accumulated in the inflamed ear, while practically none were seen in the healthy normal ear on the other side or other organs in the body. Guiding CargocytesTM to the right location was now a very real possibility.
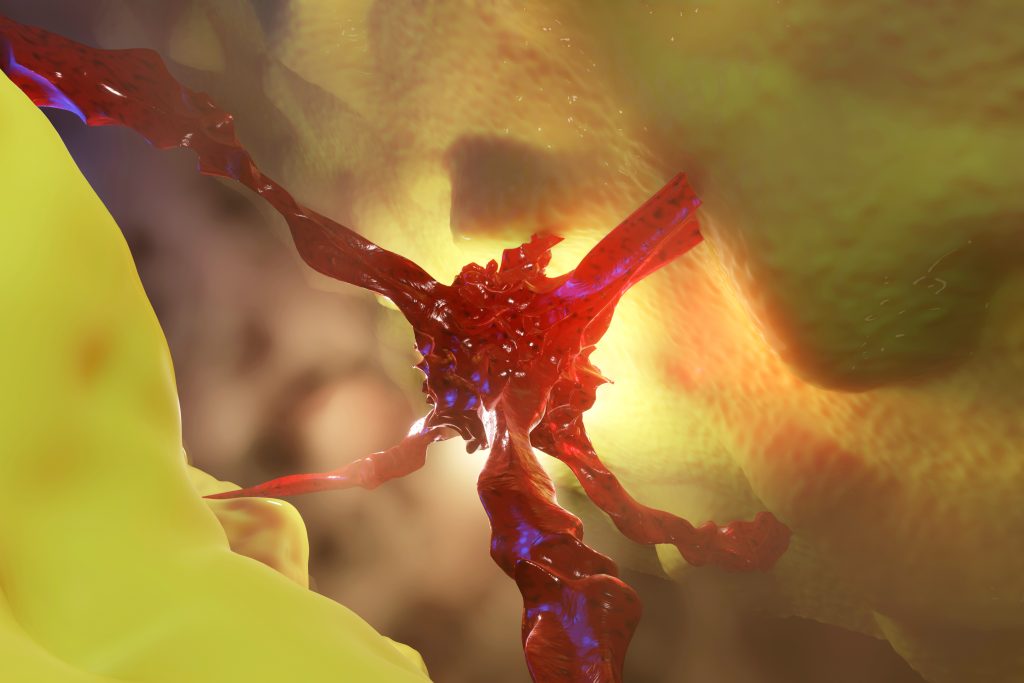
Cancer on healthy tissue.
Loading the Cargo
Finally, Professor Klemke and his team introduced a third modification in which they inserted a purified mRNA that made the CargocytesTM produce IL-10, a strong anti-inflammatory molecule. When these triple-modified CargocytesTM were injected into mice with one inflamed ear, their homing to the site of the inflammation worked as expected.
Furthermore, due to the action of IL-10, the swelling of the inflamed ear resolved rapidly. An even more rigorous preclinical test of the triple-modified CargocytesTM involved tests in animals with inflammation of the pancreas. Sitting deep behind the stomach and hence difficult to approach even during surgery, the pancreas produces digestive juices and insulin. The inflammation of this vital organ is a common life-threatening problem in humans. Experiments demonstrated that triple-modified CargocytesTM found their way to the damaged gland and the anti-inflammatory effect of IL-10 significantly reduced further damage to the pancreas in the treated mice. Furthermore, the team did not find any evidence of adverse effects associated with the administration of CargocytesTM, instilling further optimism.
Not Perfect – Yet…
These results are extremely encouraging. They show that CargocytesTM represent a novel and highly promising way to selectively deliver drugs to specific locations. This possibility will open up important new therapeutic approaches although challenges remain. First of all, production and manufacturing methods need to be scaled for clinical applications and the safety of CargocytesTM needs to be rigorously tested before clinical use. Professor Klemke’s team will also need to show that by changing the chemokine receptors and adhesion molecules, they can direct CargocytesTM to other locations, for example, to a tumour.
This dedicated work means that Professor Klemke is well on the way to making science fiction a reality. In the not-too-distant future, these tiny little biological structures may be able to improve the medical treatment of millions of people.
SHARE
DOWNLOAD E-BOOK
LISTEN TO THE AUDIO
REFERENCE
https://doi.org/10.33548/SCIENTIA833
MEET THE RESEARCHER
Professor Richard L. Klemke
University of California San Diego
San Diego, CA
Professor Richard Klemke earned his PhD in Cell and Developmental Biology from Texas Tech University Health Sciences Center in the USA. After working as a research scientist at Scripps Research (previously known as The Scripps Research Institute), he joined the University of California San Diego’s Moores Cancer Center as Professor of Pathology in 2006, where he is now Professor of Pathology and Cancer Biology. Over the past 20 years, Professor Klemke has been a highly active teacher, mentor and lecturer in the academic realm, and also a valued consultant in the biotech and pharmaceutical industries. His laboratory has received numerous federal and private awards to support his development of novel therapeutics for cancer and investigations into the critical mechanisms that drive cancer progression and cancer immunity. Unsurprisingly, Professor Klemke’s pioneering work has attracted international recognition, and he continues to provide new insights into the underlying processes of how malignant tumours develop, induce a blood supply, and avoid immune recognition.
CONTACT
FUNDING
National Institutes of Health grants (R01 CA097022, CA184594, CA182495, HL082792, GM137605 and U54 CA210184)
National Science Foundation (CAREER Award CBET-1254846)
Hartwell Foundation
Center for Drug Discovery and National Institutes of Environmental Health Science Grant 5R25ES020720-09.
Center for Drug Discovery and Innovation at University of California San Diego
FURTHER READING
H Wang, CN Alarcon, B Liu, et al., Genetically engineered and enucleated human mesenchymal stromal cells for the targeted delivery of therapeutics to diseased tissue, Nature Biomedical Engineering, 2021. DOI: https://doi.org/10.1038/s41551-021-00815-9.
JW Yoo, DJ Irvine, DE Discher, S Mitragotri, Bio-inspired, bioengineered and biomimetic drug delivery carriers, Nature Reviews in Drug Discovery, 2011, 10, 521–535. DOI: https://doi.org/10.1038/nrd3499
TM Allen, PR Cullis, Drug delivery systems: entering the mainstream, Science, 2004, 303, 1818–1822. DOI: https://doi.org/10.1126/science.1095833
REPUBLISH OUR ARTICLES
We encourage all formats of sharing and republishing of our articles. Whether you want to host on your website, publication or blog, we welcome this. Find out more
Creative Commons Licence (CC BY 4.0)
This work is licensed under a Creative Commons Attribution 4.0 International License.
What does this mean?
Share: You can copy and redistribute the material in any medium or format
Adapt: You can change, and build upon the material for any purpose, even commercially.
Credit: You must give appropriate credit, provide a link to the license, and indicate if changes were made.
SUBSCRIBE NOW
Follow Us
MORE ARTICLES YOU MAY LIKE
Stroke: A Global Challenge in Need of Innovation
Stroke is the third leading cause of both death and disability worldwide, but unfortunately, current diagnostic tools are unsatisfactory. Dr Roustem Miftahof and Dr Alexander Hermann at Advanced Biosimulation Technologies LLC, USA, are overcoming the limitations of existing diagnostic tools with the development of the Neuro-Glia-Vascular Unit Engine. This transformative tool for clinicians and biomedical researchers provides patient-specific health insights with the potential to improve care not only in stroke but across a range of neurological disorders.
Dr Alyson J McGregor | Uncovering the Gender Gap in Medical Research: How Sex Differences Impact Healthcare Outcomes
Medical research has historically focused predominantly on male subjects, leading to dangerous gaps in our understanding of how diseases and treatments affect women. Dr Alyson McGregor from University of South Carolina School of Medicine Greenville has devoted her career to addressing this critical issue, highlighting how biological sex differences impact health outcomes. Her work demonstrates that ignoring these differences can have life-threatening consequences and advocates for more inclusive research practices to ensure safe and effective healthcare for everyone.
How Food Environments Shape Our Eating Habits
How we eat dramatically impacts our health, yet millions of Americans live in ‘food deserts’ – areas with limited access to fresh, nutritious food. Recent research reveals that solving this crisis requires looking beyond just physical access to food to understand how our entire community environment shapes our dietary choices. Through a series of pioneering studies, Dr Terrence Thomas and colleagues at North Carolina A&T State University have been investigating how different aspects of our food environment influence what we put on our plates. Their findings suggest that creating lasting change requires reimagining how communities engage with food at every level.
Dr Jim Wu | Ziresovir Offers New Hope for Treating Respiratory Syncytial Virus Infections
Respiratory syncytial virus (RSV) causes respiratory tract infections in children and adults. While for many patients the outcomes of infection are mild, for others, infection can prove fatal, and there is a lack of effective treatments. Dr Jim Wu from the Shanghai Ark Biopharmaceutical Company in China carries out his vital research to develop new, safe, and effective treatments to tackle this killer.