Dr Irina S. Druzhinina – Using Mother Nature to Help Clean Up Mother Nature
Mycologist and Environmental Engineer Dr Irina Druzhinina and her colleagues at the Biochemical Technology Research Division of the Institute of Chemical, Environmental and Biological Engineering, TU Wien in Vienna, Austria, are looking for a compound to use in the disposal of plastic waste material to prevent environmental pollution. They think this substance may have already been invented – by a naturally occurring fungus.
Dealing with the Unnatural Naturally
‘Plastics’ – that word was one of the top 100 movie quotes of all time, from the classic film The Graduate. The advice given to graduating Benjamin Braddock, played by young Dustin Hoffman, was that there was a great future in plastics. And after World War II, this was certainly correct. The technological explosion of knowledge in the petrochemical industry has had a profound effect on our lives. You’re probably wearing clothing made out of polyester, such as Terylene or Dacron. You may be drinking bottled water or soda from a plastic bottle. And you may live in a building with insulation or other components made of Mylar. All of these substances are forms of polyethylene terephthalate, or PET – polymerised units of the monomer ethylene terephthalate. This ubiquitous material is the fourth most common plastic in the world today, following polyethylene, polypropylene and polyvinyl chloride. And as just about every news article on environmental pollution will tell you, such refuse as discarded plastic bottles are the bane of conservationists the world over. What are we to do with all that discarded plastic? It may be that Mother Nature already has that figured out, at least to some extent.
Although PET is so familiar to us, in an evolutionary sense it is a newcomer on our planet. The natural mechanisms for its recycling may evolve in future, like what has happened for cellulose, for example, but this process is very slow and takes millions of years. For now, PET is not easily biodegradable and we, humans, are in charge for its recycling. However, there are naturally occurring polyesters that can be degraded by microorganisms. The plant cuticle – the hydrocarbon, lipid and wax film that covers the outer surfaces of leaves to protect it from external invasion and to prevent water loss by evaporation – is a type of polyester, generically called cutin. And as you might expect, there are naturally occurring enzymes that can break down cutin. Some species of fungi and bacteria make cutinases that are able to hydrolyse cutin, so that the fungus can invade the plant’s leaves and basically enjoy a good salad. After all, microbes have to eat, too. Of course, cutin is not a plastic. But the mechanism to degrade polyesters clearly exists in nature – it’s just for scientists to find a way to make it applicable to artificial polyesters like PET. Dr Druzhinina and her colleagues do extensive research on that very question.
Knowing a Fungus Inside and Out
Dr Druzhinina has studied a particular fungus for over 15 years – the genus Trichoderma. Trichoderma is employed in industry to produce cellulolytic enzymes. Various species of this fungus are known as environmental opportunists, as they are common in a diversity of habitats such as soil, other fungi, dead wood and living plants, the world over. Trichoderma is also used as a biofungicide and biofertiliser for plant protection in organic agriculture, being widely considered as a plant-beneficial fungus.
Since these fungi are known to be extremely industrially important, they have been studied extensively. Therefore, much is known about their physiology, genetics and the various enzymes and chemicals they produce. Dr Druzhinina herself has published a number of papers dealing with the evolution, physiology, ecology and genetics of Trichoderma. In fact, in 2011 Dr Druzhinina and colleagues published an article in the Nature Reviews Microbiology that clarified the ecological genomics of Trichoderma fungi by integrating the knowledge on the genomes of some species with physiological and ecological characteristics of a wide array of species. Clearly, Dr Druzhinina has become quite an expert on the fungus Trichoderma. This put her in an excellent position to see a possible extension for Trichoderma to other branches of biotechnology, such as the plastics pollution problem.
There’s Degradation and Then There’s Degradation
Getting rid of plastics is not an easy thing to do. Ideally, enzymatic recycling of polymers and especially of PET would separate the polymer into its constituent pieces, namely ethylene glycol and terephthalic acid. These compounds are pretty valuable and can be reused in a variety of chemical processes, including the production of more PET. Currently, however, PET recycling either requires pure PET fractions – which is difficult practically, because of all the leftover contaminants we throw away with our bottles and other trash – or you have to deal with the contaminants, which complicates the process. Also, one reason PET is so useful in bottles and other manufactured materials is that it repels water, or is hydrophobic. In order to recycle it, the PET has to be modified to increase its hydrophilicity – its ‘wettability’. Synthetic polymers such as PET also show excellent chemical resistance, but this feature makes them very difficult to mix with various chemicals to degrade them, and poses a big hurdle in the degradation process. Currently you need strong treatments, such as the use of harsh chemicals like concentrated acid or alkali or different types of plasma furnaces, to add reactive chemical groups to the PET molecules to cause degradation. It is no surprise, then, that enzymatic degradation has recently received considerable attention. Using enzymes to degrade PET is presumably more environmentally friendly than current techniques. No harsh acids or alkalis, not to mention hot plasma furnaces, are needed if you use enzymes that work near room temperature.
A number of different enzymes are already known that can degrade PET to some extent. Among them, cutinases seem to be the most effective. There is one problem, however – the process is slow. Apparently PET is so hydrophobic that some enzymes have trouble ‘sticking’ to the PET to get the job done. Regular plant cutin isn’t so tough, but PET was designed this way. Thankfully, however, scientists have described another type of substance that can help here – small secreted cysteine-rich proteins called hydrophobins. These are unique proteins produced by fungi. Threads of fungi (called filaments or hyphae) develop either on the substrate that the fungus is eating or directly in it. This means that the good attachment is a critical function for the survival of the fungus. Here hydrophobins help: they are amphiphilic and naturally adhere to both hydrophobic and hydrophilic surfaces changing their property towards the opposite. In other words, hydrophobins can stick a fungus or an enzyme practically to any surface including the hydrophobic PET, allowing the enzyme to do the work of degrading the PET.
This is where Dr Druzhinina is applying her intimate knowledge of Trichoderma. Interestingly, species of Trichoderma that are particularly opportunistic happen to have an unusually high number of genes that code for the hydrophobins. Moreover, each species seems to have its unique set of these proteins that for several hundred Trichoderma species creates a treasury of surface-active proteins with potentially useful properties.
‘This is a unique property of Trichoderma,’ Dr Druzhinina tells us. ‘We already know the functions of some hydrophobins but we also need to understand why so many of them are needed for the daily life of the fungus.’ She and her colleagues had already characterised several novel hydrophobins and also revealed evolutionary mechanisms that have resulted in the outstanding diversity of hydrophobin-encoding genes in Trichoderma species. She knew all about Trichoderma hydrophobins and this challenge of looking for their ability to change properties of hydrophobic surfaces including plastics was right up her alley.
Not One Magic Bullet, but Many!
Dr Druzhinina and her co-investigators started with the idea that hydrophobins could possibly be used to modify PET surface in order to make it more accessible for enzymes. They looked at a diversity of Trichoderma species for possible hydrophobins and found several types that nobody had described before. These hydrophobins proved to be extremely difficult to handle, due to them being very surface active, or ‘sticky’, and small. Therefore, the team then spent several years developing systems to produce these hydrophobins in both bacteria and fungi – in what the biotechnologists refer to as their ‘cell factories’. ‘Agnes Przylucka, who researched hydrophobin production in the course of her PhD thesis, found that the properties of hydrophobins made in different systems – in yeasts, in moulds or in bacteria – are essentially different. All systems allow us to obtain active proteins but their good and bad properties – both are based on amphiphilicity – vary depending on the platform,’ Dr Druzhinina explains. The team developed a pipeline for the characterisation of hydrophobin properties based on their physicalchemical methods and computer modelling.
In addition to investigating natural diversity of hydrophobins, Dr Gunseli Bayram Akcapinar, who spent her two-years sabbatical in TU Wien working on hydrophobins, inspired the team to establish the ‘rational design of hydrophobins’, i.e. to engineer new versions of these compounds in the lab. ‘We looked at hydrophobins in evolutionary perspective, modelled their structure, and then, based on this knowledge, we decided which amino acids should be replaced in order to get more surface active hydrophobins,’ Dr Bayram Ackapinar explains. ‘With this approach we may achieve a kind of fine tuning of a particular hydrophobin for a particular polymer/enzyme combination.’ These rationally designed hydrophobins were then also produced in the team’s cell factories and tested for the ability to interact with an array of synthetic polymers including Mylar, Capton and PET.
To allow the team visualise how hydrophobins bind to surfaces, other PhD students of Dr Druzhinina, Feng Cai and Tatyana Yemelyanova, engineered fluorescent hydrophobins in order to see how they behave under natural conditions such as on plant roots or other fungi, and on glass surface and synthetic polymers.
From their research, Dr Druzhinina and her colleagues can see that although some hydrophobins can aid biodegradation of PET, the interaction between them and various Trichoderma enzymes is a way more complex than initially thought. Interestingly, the understanding of the physical and chemical properties of individual hydrophobins helps to predict their function in the fungus, i.e. in the natural habitat. In turn, when the function of the protein is known, the search for the proteins with specific desired function becomes more rational. This means the team continues their work by expanding their knowledge on Trichoderma hydrophobins and on ecological genomics of this fungus and on other fungi in its dwelling.
Perspective: Searching for the Right Microbes in the Right Place
Hydrophobins, however, do not perform the degradation, but only aid the enzyme. The team has an idea that the leaves of gigantic tropical trees with thick cutin layers is the right place to search for microorganisms that are capable of degrading plastics. The phyllosphere, or aerial parts of plants, is an ecosystem that hosts a large and diverse microbial community. ‘The adaptation of these microorganisms to their habitat requires the development of specialised functions such as efficient attachment to the leaf surface, resistance to oxidative stress and survival in an oligotrophic environment,’ explains Dr Marica Grujic, a biochemist working in Dr Druzhinina’s lab. To solve these challenges, phyllosphere microorganisms produce enzymes that hydrolyse not only lignocellulose (a major component of plant matter) but also polyester polymers such as cutin. As modern synthetic polymers such as PET and many others have structural resemblance to cutin, it can be assumed that phyllosphere microorganisms may be also capable of degrading plastic waste. Microbiologist Mohammad Rahimi has already isolated several hundred of microorganisms from phyllosphere. Some of these fungi are promising for plastic degradation. ‘We hope to find both: novel hydrophobins or novel enzymes such as cutinases or other enzymes that may degrade not only plant biomass but also synthetic polymers,’ Dr Druzhinina explains.
By this integrative approach, they also hope to find other types of hydrophobins or similar proteins that might be useful for industrial applications, where modification of surface properties is important: creating emulsions, coating plastic nanoparticles for drug delivery, and of course, degrading PET. That way instead of fouling up the scenery and polluting the rivers, they can be broken down and recycled to make more bottles and clothing for all of us.
Meet the researcher
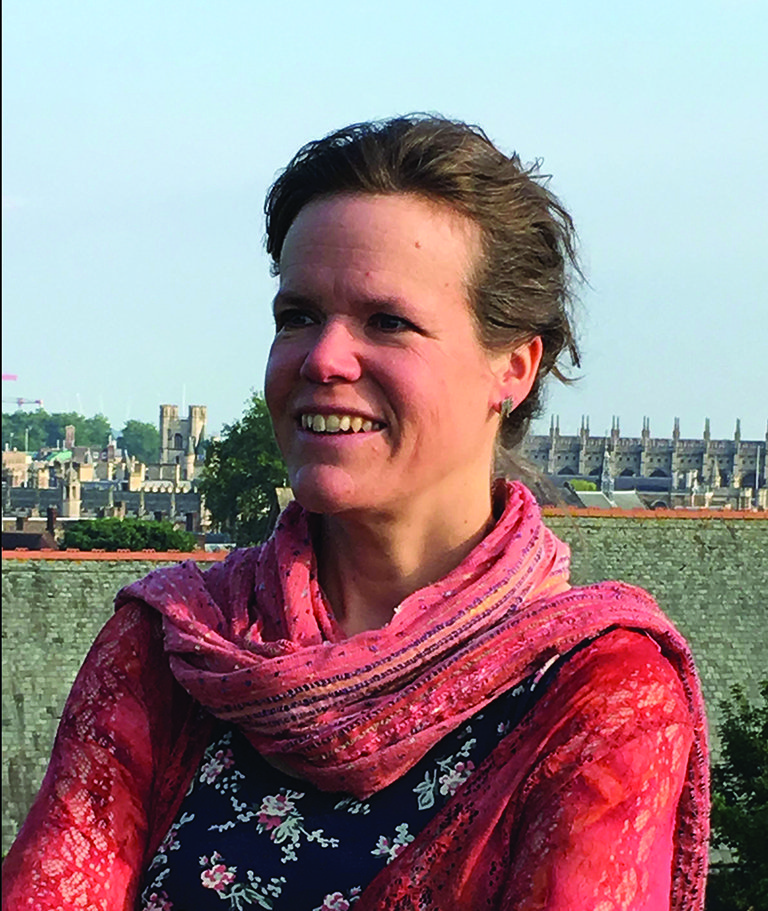
Dr Irina S. Druzhinina
Associate Professor Biochemical Technology Research Division Institute of Chemical,
Environmental and Biological Engineering
TU Wien,
Austria
Dr Irina Druzhinina received her background education in mycology in Moscow State University in Russia and pursued her doctoral studies at the Institute of Botany, University of Vienna, where she was awarded her Doctorate of Natural Sciences in 2001. After her training, Dr Druzhinina joined the faculty of the Institute of Chemical, Environmental and Biological Engineering at TU Wien, Vienna, where she is currently Associate Professor leading the Microbiology group in the research division of Biochemical Technology.
Dr Druzhinina’s research interests include applied microbiology and biotechnology, comparative genomics and molecular evolution. She has authored or co-authored over 150 articles published in peerreviewed journals and other professional proceedings. She lectures in and teaches microbiology, genomics, bioinformatics and molecular evolution. She also has developed online courses in protein phylogeny and an on-line laboratory on applied bioinformatics.
CONTACT
T: (+43) 1 58801 166551
E: irina.druzhinina@tuwien.ac.at
W: http://www.vt.tuwien.ac.at/biochemische_technologie/mikrobiologie/
Scientific papers of Druzhinina’s group on hydrophobins may be found in NCBI PUBMED https://www.ncbi.nlm.nih.gov/pubmed/?term=druzhinina%20hydrophobins
KEY COLLABORATORS
Dr Gunseli Bayram Akcapinar, Acibadem University, Turkey
Professor Erik Reimhult, University of Natural Resources and Life Sciences, Austria
Professor Hinrich Grothe, Institute of Materials Chemistry, Austria
Professor Qirong Shen, Nanjing Agricultural University, China
HYDROPHOBIN TEAM IN TU WIEN
Feng Cai
Komal Chenthamara
Marica Grujic
Alexey Kopchinskiy
Victor Lobanov
Agnieszka Przylucka
Mohammad Rahimi
Tatyana Yemelyanova
FUNDING
Austrian Science Fund (FWF)
Vienna Science and Technology Fund (WWTF)
Austrian Centre Industrial Biotechnology Carbios China Scholarship Council (CSC)
The Scientific and Technological Research Council of Turkey (TUBITAK)
REFERENCES
M Rodriguez-Mora, M Scranton, GT Taylor, A Chistoserdov, The dynamics of the bacterial diversity in the redox transition and anoxic zones of the Cariaco Basin assessed by massively parallel tag sequencing, FEMS Microbiol. Ecol., 2015, 91. doi: 10.1093/femsec/fiv088
MI Scranton, GT Taylor, R Thunell, C Benitez-Nelson, F Muller-Karger, K Fanning, L Lorenzoni, E Montes, R Varela, Y Astor, Interannual and decadal variability in the nutrient geochemistry of the Cariaco Basin, Oceanography, 2014, 27, 148–159.
GT Taylor, F Muller-Karger, RC Thunell, MI Scranton, Y Astor, R Varela, L Troccoli-Ghinaglia, L Lorenzoni, KA Fanning, S Hameed, O Doherty O, Ecosystem response to global climate change in the southern Caribbean Sea, Proc. Natl. Acad. Sci. USA, 2012, 109, 19315–19320.
V Edgcomb, W Orsi, J Bunge, SO Jeon, R Christen, C Leslin, M Holder, GT Taylor, P Suarez, R Varela, S Epstein S, Protistan microbial observatory in the Cariaco Basin, Caribbean. I. Pyrosequencing vs Sanger insights into species richness, J. Int. Soc. Microb. Ecol., 2011, 5, 1344–1356.
GT Taylor, MI Scranton, M Iabichella, T-Y Ho, RC Thunell, F Muller-Karger, R Varela, Chemoautotrophy in the redox transition zone of the Cariaco Basin: A significant midwater source of organic carbon production, Limnol. Oceanogr., 2001, 46, 148–163.
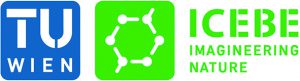