Dr. Jonathan Wright – Secrets Of Molecular Evolution In Zebrafish Genes
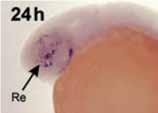
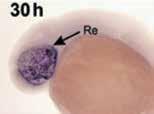

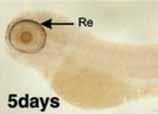
What drew you to the field of evolutionary biology?
Charles Darwin’s theory of evolution by natural selection has always been vaguely in the background of my research, but I never thought in the early years of my career that I would land in the field of evolutionary biology. As an undergraduate in Canada, I had no great interest in biology and was considering a career in music, studying classical guitar and flute. That all changed in my 3rd year of undergraduate studies when my microbiology professor invited me to start an honours research project. By the end of the summer project, I was hooked on research and decided on graduate studies. I had no career goals – I simply enjoyed research, particularly the bench work. Curiosity was my sole motivation.
My research experience has focused on defining molecular mechanisms that control gene expression in bacteria, algae and fish. My early work also involved population and behavioural studies on a range of endangered wildlife species and developing some of the first DNA fingerprinting tools for genetic studies of fish. Today, these genetic tools are still widely used in conservation, population and behavioural biology.
How can your work on zebrafish help us better understand the diversity of life on the planet, including the origins of our own species?
Most vertebrate genomes contain ~20,000+ genes and the complexity of these animal genomes are the result of at least two rounds of whole genome duplication that occurred in vertebrates well before the fish whole genome duplication. The remnants of these two previous whole genome duplications in early vertebrates are barely detectable – the gene sequences have changed so much that duplicate genes are barely recognized as twins, if at all. Our work offers an opportunity to define molecular evolutionary processes that forged the structure of extant vertebrate genomes, including the human genome, in the crucible of natural selection.
What are the possible implications of your work for human medicine?
A class of regulatory proteins, now known to control the differential expression of vital gene groups in teleost fishes, has received increasing attention from the pharmaceutical industry. These peroxisome proliferatoractivated receptors (PPARs) have been implicated in myriad human diseases such as hypercholesterolemia, obesity, arthritic inflammation, and diabetes. Currently, PPARs are under intensive study as targets for therapeutic treatment of these debilitating human afflictions.
What do you believe is the most significant outcome from your research career?
The students I have trained. I hope I’ve imparted to them the delight of curiosity, exploration, and an understanding of how scientific research is done. I hope they enjoyed the human aspect of science, the exchange of ideas, and playful discussions. I hope, too, they will pass these qualities on to their own students.
What is your assessment of the current state of scientific research funding? What legislation or policies would you change to improve how science in your field is done?
The general public and our elected representatives in government often view scientific research as a means to economic growth. This leads national science funding agencies to prioritize applied science leading to technological innovation while discovery science is increasingly underfunded. I think this is a mistake, as we cannot always predict where fundamental, discovery-based research will lead us. Science, like all of the arts, is an intrinsically human activity of seeking knowledge about the universe and ourselves. I believe few would argue against civic support to art museums or ballet companies. I ask myself, perhaps naively, why is ‘curiosity-driven’ science not government-funded simply for its intrinsic value to humanity? On rare occasions, scientific discovery leads to profound insight into the workings of the universe. New discoveries may also lead to creation of leadingedge industries generating enormous wealth or wondrous health benefits, or both. Let me briefly illustrate this point with one example: In the 1970’s Werner Arber, Daniel Nathans and Hamilton O. Smith were independently studying DNA modifying enzymes in bacteria. In today’s funding climate, this work might receive scant attention. But Arber, Nathans and Smith shared the Nobel Prize in Physiology or Medicine in 1978 for their discovery of restriction endonucleases, enzymes that cleave DNA at specific nucleotide sequences. These essential tools enabled the development of recombinant DNA technology, which in turn led to today’s $400 billion dollar biotechnology industry.
The Evolution of Genetic Light Switches
Over 150 years after Darwin’s seminal ‘On the Origin of Species’ was first published, biologists are still defining the molecular underpinnings of evolution. Dr. Jonathan Wright’s zebrafish studies give us insights into how the mechanisms that turn genes on and off may have evolved in our own species.
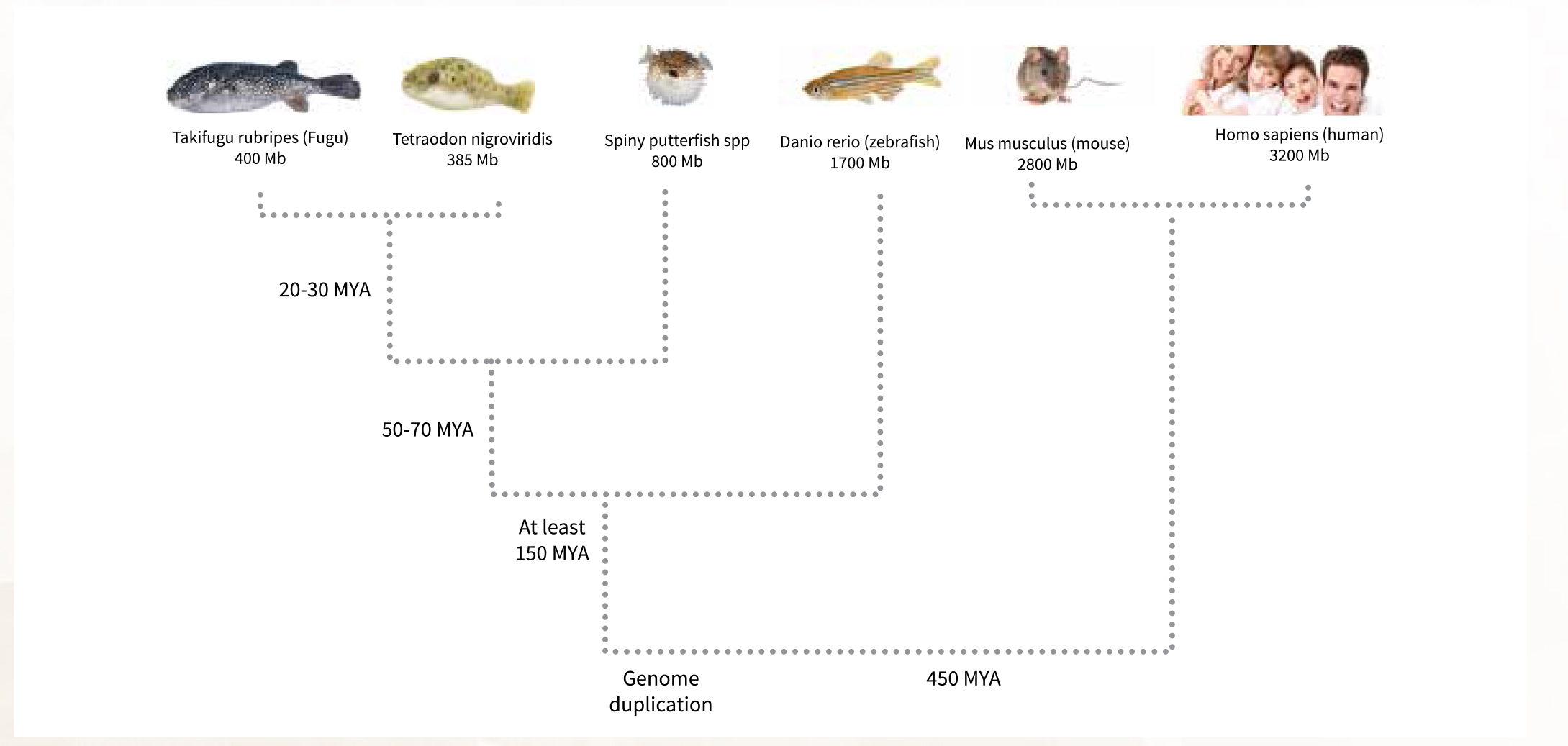
ALL EVOLUTION IS MOLECULAR
On childhood fishing trips in England, Wright walked in Charles Darwin’s footsteps around the naturalist’s family lake at Maer Hall in Staffordshire, England. Perhaps it was that early brush with the great theorist that ultimately drew Wright, an aspiring musician, to study the molecular machinery of evolution.
The abstract notion of a gene as the fundamental unit of heredity was not coined until nearly 30 years after Darwin’s death. Today, biologists know that evolution is rooted in molecular changes of genes. Genes are stretches of DNA, some of which acts as a blueprint a cell can read, transcribe into RNA and in turn translate into protein, generating the phenotype of an individual organism. Other stretches of ‘non-coding’ DNA do not contain protein recipes but instead interact with certain proteins that make nearby stretches of coding DNA either available or inaccessible to the cellular machinery. The result: portions of an organism’s genetic blueprint is either closed (gene turned off), or opened to be read (gene turned on).
DNA sequence mutations in sperm and egg cells The Evolution of Genetic Light Switches Over 150 years after Darwin’s seminal ‘On the Origin of Species’ was first published, biologists are still defining the molecular underpinnings of evolution. Dr. Jonathan Wright’s zebrafish studies give us insights into how the mechanisms that turn genes on and off may have evolved in our own species. happen by exposure to environmental factors (such as, UV radiation in sunlight or natural or human-made chemicals), or spontaneously occur by random errors during DNA replication, processes that fuel the engines of evolution. When an essential part of the DNA is mutated, it will be removed from the gene pool through the pressures of natural selection. Mutations with adaptive benefit will spread throughout the population.
On very rare occasions, DNA mutation leaves little room for permanent inheritable changes in the genetic code with the catastrophic consequence of a lethal genetic disorder in the offspring of the next generation. Sometimes, however, mutation provides more raw material for genetic innovation. Before a reproductive cell divides and proliferates, it must create a full copy of its genetic material to pass on to each daughter cell. If this duplication proceeds abnormally, one daughter cell may retain both DNA copies. Such whole genome duplication (WGD), the doubling of an organism’s genetic information, is believed to be one of the main forces underlying the increased complexity and diversity of life on earth. Susumu Ohno, the father of gene duplication theory, proposed in the 1960s that there had been at least two What is your assessment of the current state of scientific research funding? What legislation or policies would you change to improve how science in your field is done? The general public and our elected representatives in government often view scientific research as a means to economic growth. This leads national science funding agencies to prioritize applied science leading to technological innovation while discovery science is increasingly underfunded. I think this is a mistake, as we cannot always predict where fundamental, discovery-based research will lead us. Science, like all of the arts, is an intrinsically human activity of seeking knowledge about the universe and ourselves. I believe few would argue against civic support to art museums or ballet companies. I ask myself, perhaps naively, why is ‘curiosity-driven’ science not government-funded simply for its intrinsic value to humanity? On rare occasions, scientific discovery leads to profound insight into the workings of the universe. New discoveries may also lead to creation of leadingedge industries generating enormous wealth or wondrous health benefits, or both. Let me briefly illustrate this point with one example: In the 1970’s Werner Arber, Daniel Nathans and Hamilton O. Smith were independently studying DNA modifying enzymes in bacteria. In today’s funding climate, this work might receive scant attention. But Arber, Nathans and Smith shared the Nobel Prize in Physiology or Medicine in 1978 for their discovery of restriction endonucleases, enzymes that cleave DNA at specific nucleotide sequences. These essential tools enabled the development of recombinant DNA technology, which in turn led to today’s $400 billion dollar biotechnology industry. whole genome duplication events in vertebrates leading to humans.
While working on the genetics of fish species, Wright read about the many duplicated genes present in bony fishes. Theorists assume that such a WGD over 230 million years ago led to the high numbers of duplicated genes present in modern bony fishes. Using zebrafish as an experimental model, Wright set out to investigate why so many duplicated genes were still present in the modern fish genome. Evolution does not normally permit redundancy – an extra, unnecessary copy of a gene should be lost from the genome by mutational decay. Understanding the evolution of duplicated gene pairs in bony fish could provide insights into the origin of our own species.
THE FATE OF DUPLICATED GENES
With the zebrafish genome already sequenced, Wright quickly found that the intracellular lipid-binding proteins (iLBPs) gene family had retained over 60 per cent of its duplicates. This result was striking compared to the average duplicate retention rate of 3-6 per cent across entire fish genomes. iLBP genes code for transport proteins that carry fatty acids, vitamin A, and other essential lipids around the cell. These proteins convey important signals and are essential in cellular development, growth, and reproduction. Wright hypothesized that retention of more iLBP genes could allow for the development of more sophisticated cellular signalling networks.
Researchers have long been interested in the fate of duplicated gene pairs. If the ancestral gene function is maintained by one gene copy, the other copy can serve as raw material for evolutionary adaptation, free from selective pressure. Under the prevailing model of evolution following gene duplication articulated by Force et al. in 1999, a duplicated gene has three possible fates. In many cases, it will mutate into benign uselessness, leading behind evolutionary baggage. As Wright explains, “the process of evolution proceeds by happenchance, mistakes are made, and it is often a messy process leaving behind abandoned bits of life”. Another possibility is that one copy of the duplicated gene will mutate to gain a new, adaptive function. This adaptive innovation will be preserved in the gene pool. The third possibility in Force et al.’s model is subfunctionalization, wherein each gene copy following duplication acquires through mutation only a sub-set of functions of the ancestral gene that are divided across the two duplicates. Framing his investigating with the Force’s et al. model, Wright’s curiosity about the duplicated fish genes led him to investigate how the iLBP duplicates had evolved since the teleost WGD.
REGULATING GENE EXPRESSION
Each cell in the human body contains over 20,000 genes. Yet at any moment, only a fraction of these genes will be active, readily available for DNA to be transcribed into RNA and then translated into protein. Gene expression, the switching on and off of gene activity, makes us who we are. Regulatory regions of the gene act as light switches, interacting with cellular signals to keep genes open or closed to the cell’s transcription machinery. These genetic switches are sensitive to many factors, from the cellular environment to the organism’s stages of growth and development.
Wright focused his evolutionary inquiry on regulatory regions of the iLBP genes and determined that the mechanisms that control many of these genes have diverged since the teleost WGD. Zebrafish iLBP genes appear to have developed different on-and-off switches that allow nearby coding regions to be activated at different times and in different body tissues. For example, one member of a duplicated fatty acid-binding protein gene pair is expressed in the zebrafish brain during development while the duplicate gene is expressed in the zebrafish swim bladder.
Wright’s research reveals that dietary fatty acids have different effects on iLBP genes turning them on or off at the right time when needed whether in the brain, the heart, or the liver. The complexity of the iLBP multigene family allows for the essential transport and intracellular signalling of lipids by these proteins at stages of embryo or larval development, or under environmental circumstances that fit the zebrafish’s changing needs.
NEW MOLECULAR MECHANISMS
Wright is currently working to define the precise molecular mechanisms controlling iLBP gene expression in different tissues. Recent studies have identified regulatory proteins called peroxisome proliferator-activated receptors (PPARs), which bind to specific response elements (PPREs) in the zebrafish iLBP genes. The binding action between PPARs and PPREs stimulates gene expression, opening up nearby genes to the cellular transcription machinery. Wright and colleagues seek to identify other proteins involved in the complex iLBP gene expression signalling pathway to understand how these interactions give rise to differential gene expression in different tissues at different times.
HEALTHCARE APPLICATIONS
Activities in the regulatory regions of genome appear to play a large role in common diseases such as cancer, diabetes, Parkinson’s, and Alzheimer’s. The PPARs that Wright is investigating in the context of zebrafish duplicate gene expression are currently under investigation as potential drug targets for the treatment of a range of human diseases. As research continues into understanding the cascade of molecular signalling events surrounding PPAR-meditated gene expression, Wright believes zebrafish can serve as an excellent experimental animal model. Elucidating these gene expression pathways and their behavior in diseased states could lead to the development of drugs that correct genetic dysregulation in many devastating human conditions.
Meet the researcher

Dr. Jonathan Wright
Professor
Dalhousie University
Department of Biology
Dr. Jonathan Wright received his PhD from Memorial University of Newfoundland. He studies gene and genome evolution with emphasis on the genesis and fate of duplicated genes. Dr. Wright’s research deals with the mechanisms and evolution of gene regulation in zebrafish and other fishes, focusing on the multigene family of intracellular fatty acid- and retinoid-binding proteins. Among his many awards, Dr. Wright has received four UNESCO Biotechnology fellowships, has been a visiting research scientist at universities in Stirling, Scotland, Milan and Rome, Italy, and São Paulo State, Brazil and currently serves on the advisory board of the journal, Neotropical Ichthyology. He lectures on molecular biology and genetics at Dalhousie University.
CONTACT
T: +1 902 494 6468
KEY COLLABORATORS:
Mehtap Bayir & Abdulkadir Bayir, Ataturk University, Turkey
Eileen Denovan-Wright, Dalhousie University, Canada
Allyse Ferrara & Quenton Fontenot, Nicholls State University, Louisiana, USA
Christine Thisse & Bernard Thisse, University of Virginia Health Sciences Center, USA
Santosh P. Lall, Institute of Marine Biosciences, National Research Council of Canada
FUNDING:
Natural Sciences & Engineering Research Council of Canada (NSERC)
The Scientific and Technological Research Council of Turkey (TÜBİTAK)


