Dr Philipp Scherer – Adiponectin: The Body’s Fat Controller
Dr Philipp Scherer’s lab has been studying the role of fat cells in our metabolism. Over the past 20 years he has helped to explain how not all fat is created equal, and that the signals it produces influence the health of every other part of the body.
Fat cells (adipocytes) are something most of us feel like we have too many of and would be happy to see the back of. They represent the net difference between our energy intake and expenditure, so in evolutionary terms, adipose tissue is extremely useful, allowing the body to adapt to the changing availability of food and giving us metabolic flexibility. However, modern diets combined with sedentary lifestyles can often cause adipose tissue to accumulate in excess, which is associated with a wide range of health problems including diabetes, heart disease and cancer. Contrary to popular belief, these cells do not simply constitute a reservoir of excess fat. Since the early 1990s, scientists have learned that these cells are far from simple, with adipocytes displaying highly active and complex metabolic response as well as secreting a multitude of signalling molecules, affecting virtually every tissue in the body.
Dr Philipp Scherer has been researching the intricate details of how adipose tissue interacts with other organs for the last 20 years. His laboratory studies (among other aspects of fat cell function) a particular molecule which is secreted exclusively by adipocytes, called adiponectin. He discovered this molecule in 1995 and since then, adiponectin has been the subject of over 10,000 scientific studies. Broadly speaking, this protein influences various metabolic processes and protects cells from inflammation and apoptosis (programmed cell death).
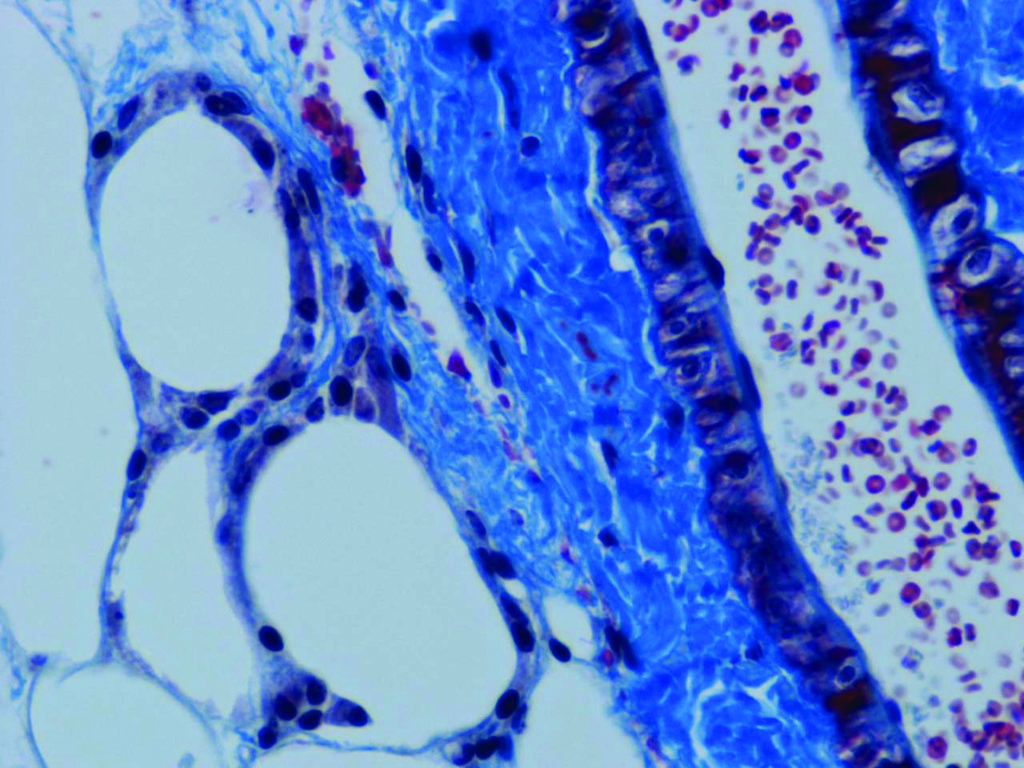
‘Despite our familiarity with fat tissue, there is a huge cloud of mystery around it’
Adiponectin, fat cells and metabolic health
Despite the specific nature of its fat-cell source, if you thought more fat means more adiponectin in the blood, you would be wrong. Counter-intuitively, the more fat you have, the less adiponectin will be in your system. In fact, adiponectin levels are negatively correlated with various metabolic disorders and diseases related to obesity. You might now be thinking, ‘great, we clearly all need some more of this!’, but again, biology often has a tendency to do the opposite to whatever common sense might suggest. When Dr Scherer’s lab used genetic engineering to produce high quantities of adiponectin, regardless of weight gain (where in an un-modified mouse, levels would decrease), they might have expected that the mice would find it more difficult to maintain the weight gain. Instead the number of adipocytes skyrocketed, producing the fattest mice ever recorded, roughly equivalent to an 800lb (363kg) human. Yet, despite the obesity, these mice were very healthy and not diabetic!
Some of these contrary observations can be explained by the fact that not all fat tissues are equal. Adipose tissue can be made up of metabolically healthy fat cells, or dysregulated and metabolically unhealthy fat cells, depending on the manner in which the tissue expands. Our metabolic health is not always related to how obese we may be, but how sensitive we are to insulin, which is a pancreas derived hormone, produced in response to high blood sugar levels. Insulin regulates blood sugar levels by signalling cells to start taking up sugar to be used for energy. Resistance to insulin makes it harder for the body to regulate blood sugar levels. This resistance develops progressively and would eventually be diagnosed as type II diabetes. As Scherer’s lab found out, insulin sensitivity is closely related to the healthiness of the adipose tissue.
As fat pads expand, blood vessels must develop with them, to provide oxygen. Hypoxia, a local insufficiency in oxygen supply, results from the failure of blood vessels to keep up with the expanding tissue, leading to various problems in its development. Hypoxia-triggered fibrosis causes increased cellular stress, and tissue necrosis (dead cells). The remnants of dead adipocytes attract white blood cells (macrophages) to clean up the mess, but this induces tissue inflammation. This inflammation, if left unresolved, leads to insulin resistance. Counterintuively, treatments aimed at reducing inflammation do not appear to have much benefit in improving sensitivity to insulin. As Dr Scherer points out, ‘adipose tissue is the biggest endocrine gland we have. It releases many protein factors, most of which are dysregulated in the obese state’. Metabolic health, the ability to tolerate high sugar or high fat diets, is therefore associated with healthy adipose tissue. The mice mentioned previously, with artificially increased adiponectin levels, displayed low levels of fibrosis in their fat tissue, as well as improved vascularisation, compared to obese, yet metabolically unhealthy mice.
White, brown and beige fat
In addition to metabolically healthy and unhealthy adipose tissue, fat cells can come in several different forms. The classical white adipocyte is the most common type of fat cell, but brown adipocytes also exist, which are specialised in heat generation and are activated by cold temperatures. A third class of adipocyte, termed ‘beige adipocytes’ are located within white fat tissue, but possess the characteristics of either white or brown fat, depending on whether they are active or dormant (activation being triggered by low temperatures). The work of Dr Scherer’s lab on adiponectin has identified a role for the protein in the differentiation of these fat cells. They have found that adiponectin is actually one of only a few signalling factors which appears capable of inducing ‘beiging’ of white adipose tissue. This may help to explain the observation that people with lower body-mass indices appear to display a higher proportion of brown fat.
Ceramides and how adiponectin influences insulin sensitivity
One of the primary functions of adiponectin appears to be sensitizing the surrounding tissues to insulin. In type I diabetic patients, for example, adiponectin levels appear to be increased, most likely to compensate for the diminished insulin production. Levels of adiponectin are also higher in metabolically healthy obese people compared to people with comparable body-mass indexes who are metabolically unhealthy.
Insulin sensitivity is impaired by a class of sphingolipids (a type of fat molecule) called ceramides, which are found in cellular membranes and block a particular part of the molecular pathway required for insulin sensitivity. Using genetically modified mice, Dr Scherer’s laboratory studied the effects of lower ceramide levels on the liver, by over-expressing acid ceramidase, an enzyme which breaks down ceramide. These mice displayed an increased sensitivity to insulin. This improvement was true not only for the liver, but for the adipose tissue as well, effectively improving insulin sensitivity throughout the whole body. In addition to insulin resistance, ceramides have been linked to inflammation and apoptosis. Another important role of adiponectin is to decrease the levels of ceramide in various tissues, including the liver and heart, and certain anti-diabetic drug treatments which produce an increased level of adiponectin appear to cause an associated decrease in ceramide levels.
Adiponectin’s tissue-specific effects
Dr Scherer’s lab has studied the influence of adiponectin in various tissues, again by using a system of genetic modifications in mice, which allow them to induce apoptosis in particular target cell types, and then study the effects of increasing adiponectin concentrations on cell survival. These mice are called ATTAC mice, for Apoptosis Through Triggered Activation of Caspase 8 (caspase being a key protein in the triggering of programmed cell death). Since adiponectin levels are inversely associated with heart attack risk and coronary heart disease, Dr Scherer’s system was used to study the effect of adiponectin on cardiac myocytes (heart muscle cells). These so-called ‘HeartATTAC’ mice show an increased rate of survival based on their levels of adiponectin. Similarly, their research shows that mice are more vulnerable to cardiac injuries if their ability to produce adiponectin is removed via genetic modification, but also in the absence of receptor molecules required for adiponectin binding to cardiomyocytes.
Heart cells are only one of many cell types that Dr Scherer’s group have studied in this way. The system was also applied to the insulin producing ß(beta) cells found in the pancreas (‘PANIC-ATTAC’ mice), which showed a similar outcome to the HeartATTAC model – increased ß cell survival with increasing levels of adiponectin. Comparable
‘Despite our familiarity with fat tissue, there is a huge cloud of mystery around it’ outcomes were also observed when they performed the study on podocyte cells of the kidney (‘POD-ATTAC’ mice), which showed improved kidney function in mice with elevated adiponectin levels.
Dr Scherer’s laboratory has shown that adiponectin not only increases insulin sensitivity, but also works with insulin to lower blood glucose levels by inhibiting glucose production by the liver. Adiponectin has a similar effect on fat production by the liver, by improving the metabolism and fat storage capabilities of adipose tissue.
‘We need to understand what the role for the different fat depots is with respect to their endocrine and paracrine contributions’
There is a strong association between detectable adiponectin in the circulation and the incidence of a number of different cancers, with low levels being linked to breast, endometrial, colon and kidney cancers, as well as certain forms of leukemia. These observations are made at the epidemiological level, looking at large numbers of people. At the cellular level, increased adiponectin can actually be associated with increased tumour growth, possibly due to its angiogenic (blood vessel growth inducing) properties, as tumours, like an expanding fat pad, require vascularisation in order to avoid hypoxia.
Due to the role of adiponectin in so many illnesses, metabolic disorders and insulin sensitivity, the receptors to which the protein binds are a source of great interest as potential drug targets, with the work of the Scherer lab and others being at the forefront of research into this area.
Uridine regulates the fasting response
One of the clearest benefits of fat tissue in the body is its ability to provide energy in times of fasting, such as by releasing free fatty acids as and when they are required. Other key nutrients are also released from adipose tissue, dependent on the metabolic situation, and one such nutrient, or metabolite, has been studied by Dr Scherer and his research group. That metabolite is called uridine, which is required for a diverse range of biochemical reactions, as well as being a key building block of RNA, which is required for the ‘reading’ of DNA. Uridine can even be used as an energy source, by neurons in particular. Normally, when we are not in a fasted state, uridine is synthesised by the liver. Dr Scherer and his team have discovered that when fasting, the liver ceases to produce uridine, which is instead provided by adipocytes, and the liver concentrates on making glucose instead, using the fatty acids released from fat tissue as an energy source.
Interestingly, uridine levels in the circulation appear to increase during fasting and decrease again after eating. Dr Scherer’s group noticed that in metabolically dysfunctional mice, uridine levels are higher in general, and that their core body temperature is a few degrees lower. These differences are even more pronounced in these mice during fasting, and it turns out that one is responsible for the other. When these mice are injected with uridine, their body temperatures drop by several degrees temporarily. Scherer suggests that fat tissue may be regulating body temperature via its uridine synthesis during fasting, when a drop in body temperature is important for reducing the metabolic rate and preserving energy. This effect appears to be entirely independent of brown or beige fat cells. The group found that glucagon, another pancreas-derived hormone with the opposite effect of insulin (it increases blood glucose levels in response to fasting), is produced when the pancreas detects high levels of uridine. Scherer suggests that taken together, these observations indicate that fat cells use uridine as a core regulator of the fasting response.
The next steps
There are still many questions to be answered regarding the role of adipose tissue on regulation of metabolism, but it is clear that functionally healthy fat is about more than just obesity. Fundamental questions about the function of adiponectin still need to be answered, such as what signals adipocytes to produce it in such high levels? Dr Scherer explains that the future work of his lab will involve ‘exploring fat tissue not only as a source of hormonal factors and lipids, but increasingly as a source for critical metabolites that the fat cell contributes to the system in times of need’. Future work will also focus on the role fat tissue has to play in cancer and other diseases. ‘’I believe we can expect many more surprising discoveries from the adipocyte in future’’, says Scherer.
Meet the researcher
Dr Philipp Scherer
Touchstone Diabetes Center
Department of Internal Medicine
University of Texas Southwestern Medical Center
Dallas, Texas, United States
Dr Philipp Scherer is Professor of Internal Medicine and Director of the Touchstone Centre for Diabetes Research at the University of Texas Southwestern Medical Centre in Dallas. Dr Scherer performed his undergraduate and graduate studies in Biology and Biochemistry at the University of Basel in Switzerland in the 1980s and 1990s. He is best known for his discovery of adiponectin in 1995 and over the course of his academic career he has published over 320 scientific papers, some of which have been cited over 1000 times. He was recently awarded the American Diabetes Association’s Banting Medal for Scientific Achievement in 2015 though his career has been distinguished with various other honours and awards.
CONTACT
E: philipp.scherer@utsouthwestern.edu
W: http://www.touchstonediabetescenter.org/schererlab/schererlab.html
KEY COLLABORATORS
Close collaborators include colleagues in the Touchstone Diabetes Centre as well as other members of the metabolism group at UT Southwestern Medical Centre, such as Drs Roger Unger, William Holland, Rana Gupta, Olga Gupta, Joel Elmquist, Jay Horton and Perry Bickel.
FUNDERS
National Institutes of Health (NIDDK)
Juvenile Diabetes Foundation (JDRF)
Cancer Prevention and Research Institute of Texas (CPRIT)
REFERENCES
P. E. Scherer, S. Williams, M. Fogliano, G. Baldini, and H. F. Lodish, A Novel Serum Protein similar to C1q, produced exclusively in Adipocytes, J. Biol. Chem., 1995, 270, 26746–26749. (This paper is the first publication on adiponectin, describing its identification and initial characterization) A. H. Berg, T. Combatsiaris, X. L. Du, M. Brownlee and P. E. Scherer, The adipocyte-secreted protein Acrp30 enhances hepatic insulin action, Nature Med., 2001, 7, 947–953. (This is the first paper describing the potent anti-diabetic effects of adiponectin)
J-Y Kim, E. van de Wall, M. Laplante, A Azzara, M. E. Trujillo, S. M. Hofmann, T. Schraw, J. L. Durand, H. Li, G. Li, L. A. Jelicks, M. F. Mehler, D. Y. Hui, Y. Deshaies, G. I. Shulman, G. J. Schwartz and P. E. Scherer, Obesity-associated improvements in metabolic profile through expansion of adipose tissue, J. Clin. Invest., 2007, 117, 2621–2637. (A conceptual leap: the first mouse model demonstrating the healthy adipose tissue expansion can overcome any negative metabolic challenge and maintain metabolic health).
W. L. Holland, R. A. Miller, Z. V. Wang, K. Sun, B. M. Barth, H. H. Bui, K. D. Davis, B. T. Bikman, N. Halberg, J. M. Rutkowski, M. R. Wade, V. M. Tenorio, M. S. Kuo, J. T. Brozinick, B. B. Zhang, M. J. Birnbaum, S. S. Summers and P. E. Scherer, The Pleiotropic Actions of Adiponectin are Initiated via Receptor-Mediated Activation of Ceramidase Activity, Nature Medicine, 2011, 17, 55–63. (This paper provides a novel mechanism of action for adiponectin, integrating all of the existing beneficial effects attributed to adiponectin, demonstrating the key anti-lipotoxic role of adiponectin by affecting the sphingolipid pathway and stimulating ceramidase activity)
J. X. Xia, W. L. Holland, C. M. Kusminski, K. Sun, A. X. Sharma, M. J. Pearson, A. J. Sifuentes, J. G. McDonald, R. Gordillo and P. E. Scherer, Targeted Induction of Ceramide Degradation Leads to Improved Systemic Metabolism and Reduced Hepatic Steatosis, Cell Metabolism, 2015, 22, 266–278. (First description that the acute lowering of ceramides in vivo improves insulin sensitivity)