Dr Sascha Preu – Illuminating Pathways to More Reliable Photoconductors
Using laser light to produce and receive high-frequency radiation, photoconductors have become a promising aspect of materials science in recent years. So far, however, their production has faced a number of barriers. Through his research, Dr Sascha Preu at TU Darmstadt in Germany has tackled many of these challenges through the use of an alloy based on indium gallium arsenide enhanced with erbium arsenide particulates, whose electrons display some intriguing properties. His team’s work has now greatly improved the prospects for photoconductors in applications including spectroscopy, imaging and non-destructive testing.
Photoconductors
Today, semiconductors are key components of some of today’s most cutting-edge technologies, as well some of the latest research into materials physics. Although their compositions are highly varied, they are united by their characteristic property of increasing in conductivity as their temperatures rise, due to the resulting behaviours of their constituent electrons.
Through over a century of intensive research into their properties, physicists have now engineered semiconductor materials with a diverse array of highly specialised properties – each suited for particular tasks. Among these relatively new classes of materials are ‘photoconductors’ – whose electron properties are influenced by the light they absorb, instead of varying temperatures.
One particular advantage of these materials is their rapid change in conductivity when they are both exposed to and removed from sources of laser light. As Dr Sascha Preu of TU Darmstadt explains, this property can be exploited to both produce and receive high frequency radiation. ‘Photoconductors are semiconductor devices that feature a high resistance, that is switched to a low resistance by a laser signal,’ he says. ‘A high-quality photoconductive material recovers the state of high resistance within less than one picosecond, which is one trillionth of a second.’
In their research, Dr Preu and his colleagues aim to improve the performance of today’s photoconductors beyond their current capabilities. As well as allowing for new techniques in spectroscopy, which they have already demonstrated in recent experiments, the team’s work could offer new improvements for applications including spectroscopy, imaging, and communications systems.
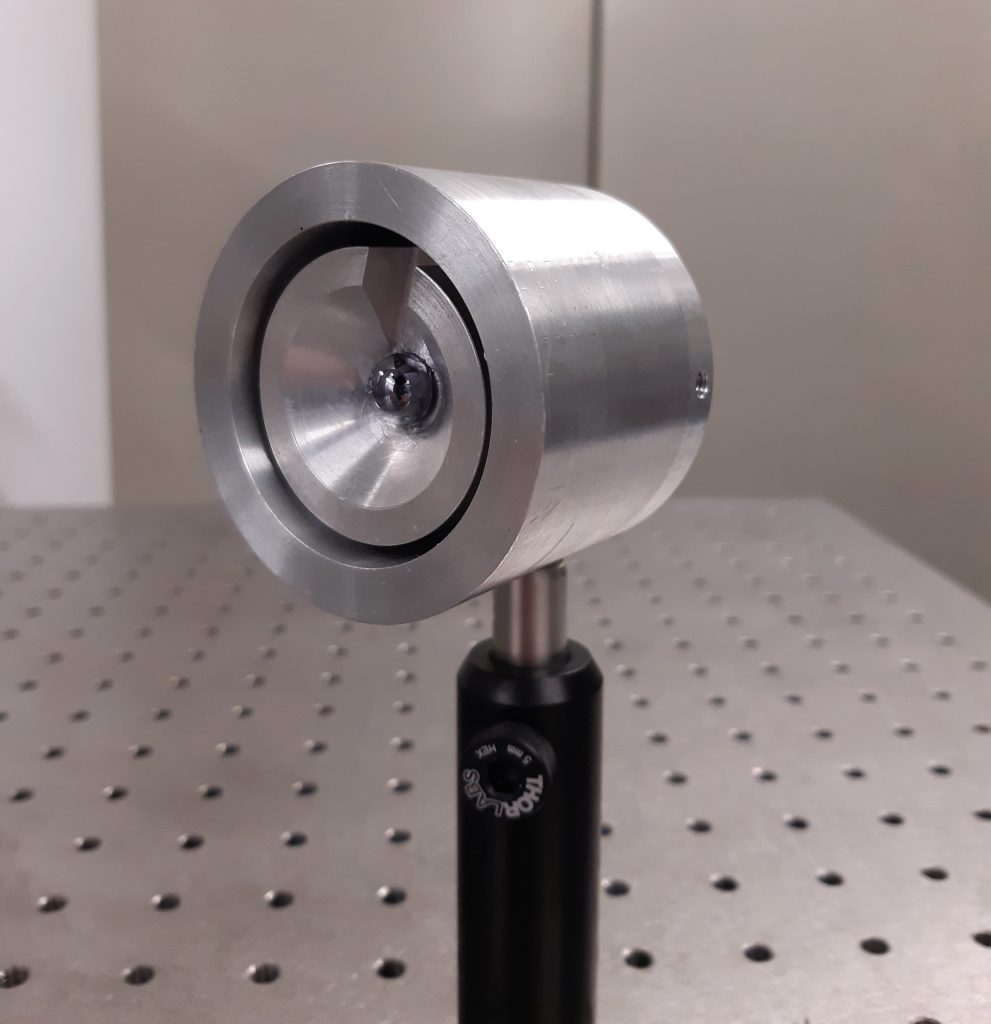
Photograph of a packaged photoconductive device
Photoconductors in High-Frequency Systems
Before photoconductors can be made to produce and receive high frequency radiation, some clever techniques are first required. In laser optics, a ‘beat signal’ describes the interference patterns that emerge between two beams with slightly different frequencies, giving a periodic variation in their collective brightness. Conveniently, the frequency of this variation is the very same as the frequency difference between the beams.
When such a beam pair strikes a photoconductor, the material’s electrons convert the beat signal into an alternating current, which changes direction at the same frequency as the frequency difference between the beams. Finally, an antenna attached to the material converts this current into ‘Terahertz’ radiation – which falls between the microwave and far infrared regions of the electromagnetic spectrum.
When driven by beat signals, these characteristics make photoconductors highly resistant to unwanted yet inevitable background noise. The inevitable background noise finally limits the minimum power that can be reliably recorded – known as ‘noise equivalent power’. Beat signals also allow photoconductors to reach high signal powers without the apparatus becoming overloaded. The ratio of the power in the system divided by the noise floor is referred to as ‘dynamic range’. Finally, they enable the materials to work within high frequency ranges.
Each of these values depends on whether the lasers are pulsed, or emitted in continuous beams. ‘Photoconductive devices play a vital role in light-dependent Terahertz systems,’ describes Dr Preu. ‘In continuous-wave systems, they serve as excellent receivers. In pulsed systems, they serve as both sources and receivers, offering frequency coverage of up to 7 Terahertz, and extremely high peak dynamic ranges beyond 100 decibels.’ Such receivers or ‘detectors’ of Terahertz radiation are invaluable in numerous technologies, including spectroscopy, astronomy and potentially communications.
Absorbing the Right Wavelength
Despite their numerous advantages, the development of reliable photoconductors is by no means an easy task for physicists. Critically, one particular wavelength of light is particularly well suited to fulfilling the potential of the materials: 1550 nanometre (nm) infrared radiation, which is a staple of many optical communications systems. While the light itself is easily attainable through commercially available laser-emitting diodes, making full use of it would prove more difficult.
To do this, Dr Preu’s team would need to construct a material which could reliably absorb the light, all while displaying just the right behaviours in its constituent ‘charge carriers’. These structures are formed when electrons are excited to higher energy levels, leaving behind a missing electron named a ‘hole’.
In a reliable photoconductor, electrons and their respective holes will move quickly when pulled along by an electric field, ensuring the highest possible conductivity when the material is illuminated. Yet at the same time, electrons must quickly fall back into their holes or engineered charge traps, and the material must become highly resistive when it is not being illuminated, ensuring the lowest possible conductivity in the dark. This way, the material can follow the modulation of the laser beat signal. ‘Further, it is challenging to engineer a material that offers high absorption at 1550 nm, sub-picosecond charge carrier lifetime, high carrier mobility, and high dark resistance – all at the same time,’ says Dr Preu.
Exploring a New Material
Since each of these properties is strongly influenced by material composition, the first major challenge for Dr Preu and his colleagues was to find a particular material which would be best suited to the task at hand.
Through studies carried out between 2010 and 2018, the researchers first experimented with a complex structure composed of erbium arsenide particulates within an indium gallium arsenide single crystal. To fabricate the material, they used a technique named ‘molecular beam epitaxy’, which is typically used in semiconductor manufacturing to deposit thin films of crystals. The films were grown by the researchers Hong Lu, Justin Norman and Arthur C Gossard at the University of Santa Barbara, California.
The resulting photoconductor, with the complex chemical formula ErAs:In(Al)GaAs, possessed many of the characteristics that Dr Preu’s team were aiming for. By demonstrating short charge carrier lifetimes, high carrier mobilities, high absorption of electromagnetic radiation at 1550 nm, and high resistance in the dark, the team could finally satisfy their initial goals.
The next step in their research would be to prove the material’s worth in real applications, particularly with respect to currently existing photoconductor technologies.
Satisfying Requirements
Having successfully fabricated their photoconductor, the researchers then rigorously tested its capabilities using theoretical models of the material. They also validated these results by comparing them to real experiments.
Through two further studies, carried out in 2017 and 2018, Dr Preu and colleagues showed for the first time that the requirements for light absorption, dark resistance, and charge carrier properties are all satisfied in ErAs:In(Al)GaAs. ‘We have demonstrated that erbium arsenide-based 1550 nm photoconductors can fulfil the aforementioned challenges in an outstanding way,’ says Dr Preu. ‘We have achieved charge carrier lifetimes around and below half a picosecond, and extremely high dark resistance. This enables frequencies beyond 6 Terahertz in pulsed operation and continuous-wave frequency ranges beyond 3 Terahertz.’
The successful demonstration of these traits through both models and experiments then paved the way for feasible considerations of how the team’s erbium arsenide-based material could be applied in real-world experiments and applications.
Applications in Spectroscopy
In a further 2019 study, Dr Preu’s team demonstrated one particularly important application for their newly fabricated photoconductor. A technique known as ‘time domain spectroscopy’ typically involves probing the behaviours of materials when they are subjected to pulses of Terahertz radiation. Just like other spectroscopic techniques, the characteristic responses of the materials can reveal many of their physical properties, including their composition, conductivity, and optical behaviours.
Currently, time domain spectroscopy has shown a clear potential for applications including quality control in industries.
The study revealed that through the team’s erbium arsenide photoconductor, pulses as short as 544 femtoseconds could be easily reached in practical telecom-wavelength operated time domain spectrometers that are both compact and inexpensive. The setup also allowed for single-shot frequency ranges as high as 4 Terahertz, and high peak dynamic ranges. All of this was made possible by the ability of ErAs:In(Al)GaAs to absorb the 1550 nm-wavelength light created by widely available laser-emitting diodes and erbium fibre lasers.
A Bright Future for Photoconductors
Through their watertight demonstrations of ErAs:In(Al)GaAs as a useful and reliable photoconductor, the findings of Dr Preu and his team have now opened up exciting new opportunities for these novel materials.
Beyond spectroscopy, their applications could include highly controllable resistors in electric circuits, accurate detectors of light intensity, and switches that operate using light instead of electric current. If applied to other situations where Terahertz radiation is commonly applied, they could be used to obtain non-invasive images of living tissues, search for concealed weapons, and transmit data.
Currently, the team applies the photoconductors within photonic vector network analysers and photonic spectrum analysers – a promising alternative to widely used electronic systems. A particular advantage of photonic systems will be the extraordinary frequency coverage in a single setup.
Through their continuing research, the researchers now hope to further improve the capabilities of low-cost photoconductor-based systems, allowing their techniques to become even more competitive in the future.
Reference
https://doi.org/10.33548/SCIENTIA473
Meet the researcher
Dr Sascha Preu
Department of Electrical Engineering and Information Technology
TU Darmstadt
Darmstadt
Germany
Dr Sascha Preu completed his doctoral degree at the Max-Planck Institute for the Science of Light and FAU Erlangen-Nuremberg in 2009. He continued his work at the University of California, Santa Barbara, as part of a Humboldt Feodor Lynen fellowship. After working as a postdoctoral associate at FAU Erlangen-Nuremberg, he became assistant professor at the Department of Electrical Engineering and Information Technology of TU Darmstadt in 2014, leading the Terahertz Devices and Systems Group. In 2018, he was promoted to a full professor. In his work, Dr Preu focuses on the development of Terahertz sources and detectors, including photoconductive materials. He also focuses on terahertz detection through specialised transistors and system-level applications, including photonic vector network analysers and photonic spectrum analysers. His research has now earned him numerous awards including the European Research Council’s ERC Starting Grant, which he received in 2017.
CONTACT
W: http://www.tsys.tu-darmstadt.de/
KEY COLLABORATORS
Arthur Gossard, University of California, Santa Barbara
Justin Norman, University of California, Santa Barbara
Hong Lu, Nanjing University
FUNDING
Deutsche Forschungsgemeinschaft (DFG), Project No. 278381540 (REPHCON)
European Research Council (ERC), Grant Agreement No. 713780 (Pho-T-Lyze)
Horizon 2020 innovative training network ‘CELTA’ Grant agreement number 675683
FURTHER READING
KH Tybussek, K Kolpatzeck, F Faridi, S Preu, JC Balzer, Terahertz Time-Domain Spectroscopy Based on Commercially Available 1550 nm Fabry–Perot Laser Diode and ErAs: In (Al) GaAs Photoconductors, Applied Sciences, 2019, 9, 2704.
AdJ Fernandez Olvera, A Roggenbuck, K Dutzi, N Vieweg, H Lu, AC Gossard, S Preu, International System of Units (SI) Traceable Noise-Equivalent Power and Responsivity Characterization of Continuous Wave ErAs: InGaAs Photoconductive Terahertz Detectors, Photonics, 2019, 6, 15.
U Nandi, JC Norman, AC Gossard, H Lu, S Preu, 1550-nm driven ErAs: In (Al) GaAs photoconductor-based terahertz time domain system with 6.5 THz bandwidth, Journal of Infrared, Millimeter, and Terahertz Waves, 2018, 39, 340.
AdJ Fernandez Olvera, H Lu, AC Gossard, S Preu, Continuous-wave 1550 nm operated terahertz system using ErAs: In (Al) GaAs photo-conductors with 52 dB dynamic range at 1 THz, Optics Express, 2017, 25, 29492.
Creative Commons Licence
(CC BY 4.0)
This work is licensed under a Creative Commons Attribution 4.0 International License.
What does this mean?
Share: You can copy and redistribute the material in any medium or format
Adapt: You can change, and build upon the material for any purpose, even commercially.
Credit: You must give appropriate credit, provide a link to the license, and indicate if changes were made.
More articles you may like
Grandmothers: Innovation Through Tradition
Grandmother Project – Change through Culture (GMP) is an organisation dedicated to documenting the role of grandmothers and demonstrating the effectiveness of grandmother-inclusive strategies in improving the health and well-being of women, children, and adolescents. GMP’s groundbreaking work challenges conventional wisdom to transform community-based interventions in Africa and beyond, harnessing a powerful but often overlooked resource: the wisdom and influence of grandmothers.
Dr Robert Larkin | Cultivating Change to Improve Soil Health and Increase Potato Yield
Environmental quality and food production are facing the pressing challenges of climate change and global population growth. Dr Robert Larkin from the United States Department of Agriculture-Agricultural Research Service (USDA-ARS) and a team of plant scientists developed and tested a range of crop management systems to help overcome these compounding challenges. Their work is improving soil health and increasing the yield of potato crops, contributing to the future food security of nations.
Professor Giorgio Buttazzo | Artificial Intelligence and a Crossroads for Humanity
Where do we stand with artificial intelligence? Might machines take over our jobs? Can machines become conscious? Might we be harmed by robots? What is the future of humanity? Professor Giorgio Buttazzo of Scuola Superiore Sant’Anna is an expert in artificial intelligence and neural networks. In a recent publication, he provides considered insights into some of the most pressing questions surrounding artificial intelligence and humanity.
Dr Ralf Adam | New Technologies Shaping the Future of Oral Hygiene
Understanding the efficiency of various toothbrush technologies is essential for achieving optimal oral health. Dr Ralf Adam, who leads a dedicated team at Procter & Gamble in Germany, is keen to investigate the complexities of these technologies. His team have provided new insights into the best toothbrush types for plaque removal and the maintenance of gum health. By highlighting the importance of informed oral care decisions and ongoing investigations, this vital research works towards ensuring everyone can achieve a brighter, healthier smile.