Dr Shantanu Basu – Exploring the Formation of Gravitationally Bound Objects Across the Universe
From brown dwarfs to supermassive black holes, many of the strangest objects known to astronomers are formed as material collapses in on itself under its own gravity. Through a combination of physical theories and the latest computer simulations, Dr Shantanu Basu at the University of Western Ontario in Canada is offering intriguing new insights into how these structures originate. His theories could help astronomers to understand the very earliest stages of bodies ranging from those too small to become stars, to the vast, all-devouring heavyweights that reside in the centres of galaxies.
From Brown Dwarfs to Black Holes
Gravity may be the weakest of the universe’s four fundamental forces, but it is nonetheless critical in shaping the landscape of the universe. In patches of space where the densities of gas, dust and other interstellar material become high enough, the force will inevitably pull it all together to form larger structures. The physical theories surrounding these processes may have been around since they were first described by Newton, but much remains to be discovered about the diverse array of bodies they can form.
This is where Dr Shantanu Basu at the University of Western Ontario comes in. ‘My overall research program is to understand the assembly of gravitationally-bound objects in the universe, whether they are stars that form in the present-day or early universe, planets or brown dwarfs that form in circumstellar disks, or supermassive black holes that form through direct collapse in the early universe,’ he explains.
Due to the sheer complexity of these scenarios, traditional calculations alone are not enough to explore them in detail. Instead, Dr Basu combines them with the capabilities of the latest simulation techniques. ‘Through the use of large computer simulations, or analytic insight where possible, our group has discovered many features of the assembly of these objects,’ he continues. With these techniques, Dr Basu and his colleagues have drawn new insights into the formation of structures including ordinary stars, bodies too small to become stars, and the very heaviest black holes in the universe.
A Star is Born
Having observed them in meticulous detail for many centuries, astronomers are now able to predict the life cycles of many types of star – from red dwarfs to blue supergiants – with high degrees of certainty. However, the very earliest stages of star formation still remain shrouded in mystery. The most widely accepted theories predict that the process occurs as interstellar material collapses, forming structures called ‘protostars’, which are too small and well hidden to observe directly.
Building on these theories, astronomers including Dr Basu use simulations to study how small, dense lumps can transform into bodies hot enough to fuse hydrogen in their cores. As Dr Basu explains: ‘Stars have been found to form through a highly episodic mass assembly process, during which a protostar can for a time brighten dramatically.’ He concludes that this process is strongly influenced by doughnut-shaped clouds of material surrounding new protostars.
Furthermore, Dr Basu proposes that some of the material in these ‘circumstellar’ disks will go on to influence planet formation, while some of it may escape the system entirely. ‘We have found that circumstellar disks can form protostellar or protoplanetary embryos that then migrate through the disk and can either fall into the central protostar, be ejected to form free-floating bodies, or carve out a gap in the disk and settle into a stable orbit,’ he continues.
In his research, Dr Basu explores these behaviours in further detail by accounting for the magnetic behaviours of fluids that conduct electricity – as is the case for circumstellar disks.
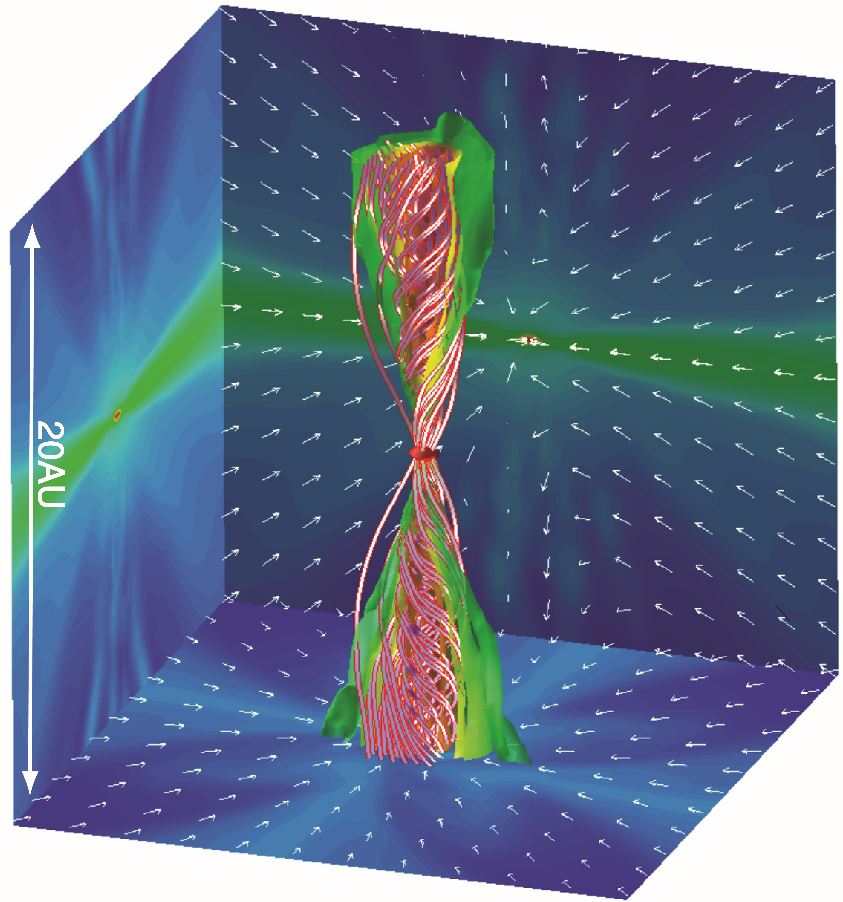
3D view of outflowing gas (green and yellow surfaces) and magnetic field lines (red lines) in the vicinity of a newly formed protostar. CREDIT: Masahiro Machida, Shantanu Basu.
Shutting Down Braking
In our own solar system, the process of ‘magnetic braking’ is a well-studied phenomenon. It occurs as electrically charged material captured by the Sun’s magnetic field rotates at the same speed as the Sun. Eventually, the material will escape, carrying with it some of the Sun’s angular momentum – subsequently slowing down its spin. Dr Basu proposes that this same effect can be seen in the environments surrounding early protostars, and initially prevents circumstellar disks from forming.
To allow disks to form, Dr Basu’s simulations suggest that this braking is shut off as the surrounding magnetic field dissipates. Furthermore, the loss in angular momentum during braking allows a hydrogen-burning stellar core to form within the disk.
‘Large scale magnetic fields are found throughout the universe, and our work shows that their removal during protostar assembly allows the formation of circumstellar disks, while also driving powerful jets and outflows of material from these regions,’ he explains.
In one of his latest studies, Dr Basu and his colleague used their simulations to follow the subsequent dynamics over the first 2,000 years after the formation of a protostar. They have now concluded that the circumstellar disk is never absent over this period; instead, it grows and its density will increase, and spiral arms will form due to gravitational instabilities. As this happens, the disk will transfer mass to the central protostar, allowing it to grow. In turn, some material flows out from the system, inducing effects including highly time-variable jets, cavities within the disk, and structures called knots and bow shocks.
Forming Failed Stars
Dr Basu also proposes that this process could explain the origins of another long-standing astronomical mystery: brown dwarfs. Typically having masses between 13 and 80 times heavier than Jupiter in our solar system, these cold objects are too large to be classed as planets, but too small to become hot enough for sustained nuclear fusion to occur. Since their initial discovery in the mid-1990s, several hundred brown dwarfs have now been observed directly. Multiple previous theories have suggested that brown dwarfs are formed as interstellar clouds collapse in on themselves directly, but currently, they disagree on the exact mechanisms by which this occurs.
As an alternative theory, Dr Basu and his colleague suggested in a 2012 study that brown dwarfs originate within circumstellar disks around protostars, in the very earliest stages of star formation. Over time, fragments of the disk will form large, dense clumps, which interact with each other through their mutual gravitational attraction. Ultimately, these dynamics will cause some fragments to gain speeds high enough to escape into interstellar space, where they finally collapse to form brown dwarfs. If this theory is correct, it would likely explain why many of the objects appear to cluster closely around young stars.
Mysteriously Colossal Structures
On far larger scales, Dr Basu has also explored the formation of the enigmatic black holes found within many galactic centres – including that of our own Milky Way. Typically, black holes are known to form when the most massive stars collapse in immense explosions called supernovae, leaving behind remnants so dense that not even light can escape from them. In the most extreme cases, these remnants can be many tens of times heavier than our Sun. However, the masses of the largest black holes known to astronomers – dubbed ‘supermassive’ black holes – are far greater, sometimes as much as a billion times heavier than the Sun.
Even accounting for how black holes will accrete mass from their surrounding environments after their formation, the laws of physics need to be stretched to allow such large structures to form at the early times in the universe’s history at which they are observed. In addition, astronomers currently remain in the dark as to why quasars – supermassive black holes with extremely bright jets originating from their poles – are so varied in their apparent brightness. Clearly, an updated physical theory is sorely needed.
Bypassing Stellar Remnants
In his latest research, Dr Basu and his student suggest that the origins of these mysterious structures don’t follow the deaths of stars, but instead lie within the universe’s earliest stages: less than 800 million years after the Big Bang. During this period, Dr Basu’s model shows that there could have been a brief era in the early universe when massive black holes formed in a way that bypassed the supernova stage entirely, instead forming directly due to the collapse of vast amounts of interstellar material under gravity. Moreover, the number of these direct collapse black holes would have been increasing rapidly during this era.
If correct, this theory could reliably explain some features of quasars, as astronomers observe them today. ‘Quasars are supermassive black holes that are swallowing prodigious amounts of material, and our work has shown that the direct collapse scenario can explain their observed luminosity distribution, and has constrained the rate and duration of their formation in the early universe,’ Dr Basu describes. ‘Using this approach, we have also been able to understand the features of the observed quasar luminosity function.’
Dr Basu’s new theory could help pinpoint the time and rate at which quasars formed in the universe. If correct, this insight would have profound implications for our knowledge of the evolution of the universe as we know it, and could be applied to understanding many of its observed features.
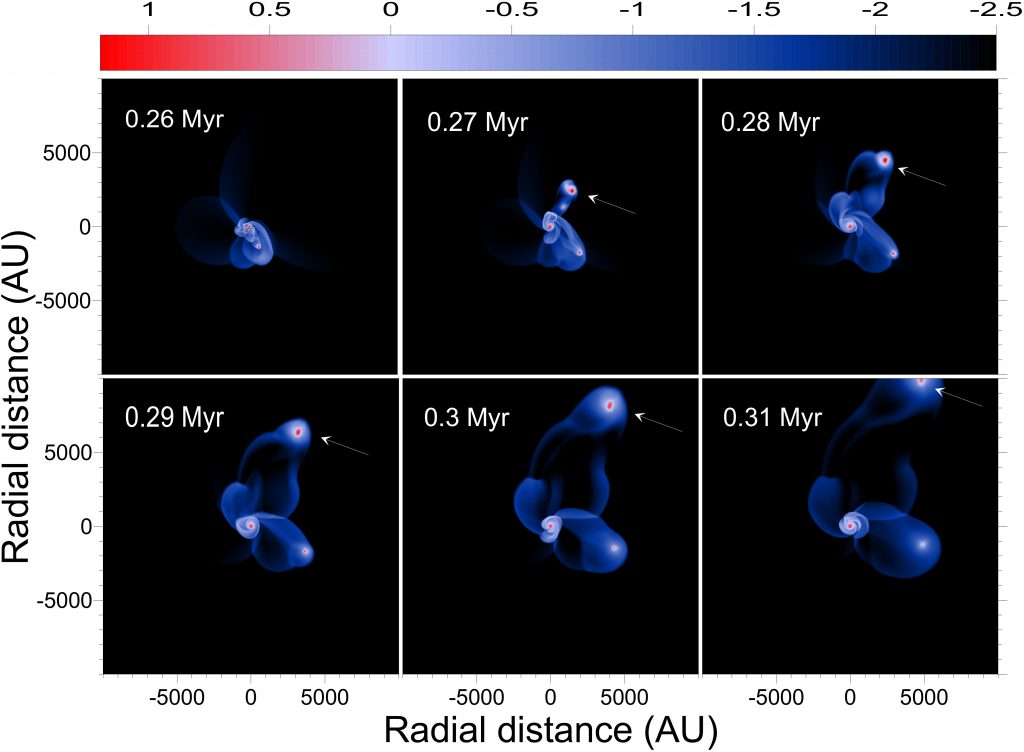
Gas surface density distribution. The arrow identifies a proto-brown-dwarf that is ejectedfrom the circumstellar disk surrounding a young protostar. CREDIT: Eduard Vorobyov, Shantanu Basu.
Understanding Gravitationally Bound Objects
As the instruments used by astronomers to observe the universe become increasingly accurate, new findings are continuing to surprise them, and throw unending curveballs towards long-established physical theories.
Yet in the face of these challenges, Dr Basu and his colleagues are pushing our understanding of the universe ever further. If their conclusions become widely accepted, astronomers could soon become far better equipped to explain the origins of the universe’s bewildering array of gravitationally bound objects.
Reference
https://doi.org/10.33548/SCIENTIA455
Meet the researcher
Dr Shantanu Basu
Department of Physics and Astronomy
University of Western Ontario
London, Ontario
Canada
Dr Shantanu Basu completed his PhD in Physics at the University of Illinois at Urbana-Champaign in 1993. He has now worked at Western since 1999, where he became a Professor in the Department of Physics and Astronomy in 2010. Dr Basu’s research interests include the physics of gravitationally bound objects: from collapsing stars and discs, to the formation of supermassive black holes in the early universe. He has made significant contributions to theories including the roles played by magnetic fields and angular momentum in gravitational collapse and star formation, as well as luminosity bursts from young stellar objects. Outside of his research, Dr Basu has demonstrated a strong involvement in international education, and has organised a number of diversity and equity-related projects in recent years.
CONTACT:
E: basu@uwo.ca
W: http://www.physics.uwo.ca/~basu/
KEY COLLABORATORS
Sayantan Auddy (ASIAA, Taiwan), Paola Caselli (MPE, Germany), Christopher Essex (UWO, Canada), Karl-Heinz Hoffmann (Chemnitz University, Germany), Takahiro Kudoh (Nagasaki University, Japan), Matthew Kunz (Princeton, USA), Sophia Lianou (National Observatory of Athens, Greece), Masahiro Machida (Kyushu University, Japan), Philip Myers (Harvard-Smithsonian Center for Astrophysics, USA), Janett Prehl (Chemnitz University, Germany), Sarah Sadavoy (Queen’s University, Canada), Yusuke Tsukamoto (Kagoshima University, Japan), Eduard Vorobyov (University of Vienna, Austria)
FUNDING
Natural Sciences and Engineering Research Council of Canada
Mitacs Canada
FURTHER READING
MN Machida, S Basu, The First Two Thousand Years of Star Formation, The Astrophysical Journal, 2019, 876, 149.
S Basu, A Das, The Mass Function of Supermassive Black Holes in the Direct-collapse Scenario, The Astrophysical Journal Letters, 2019, 879, L3.
WB Dapp, S Basu, MW Kunz, Bridging the gap: disk formation in the Class 0 phase with ambipolar diffusion and Ohmic dissipation, Astronomy & Astrophysics, 2012, 541, A35.
S Basu, EI Vorobyov, A hybrid scenario for the formation of brown dwarfs and very low mass stars, The Astrophysical Journal, 2012, 750, 30.
Creative Commons Licence
(CC BY 4.0)
This work is licensed under a Creative Commons Attribution 4.0 International License.
What does this mean?
Share: You can copy and redistribute the material in any medium or format
Adapt: You can change, and build upon the material for any purpose, even commercially.
Credit: You must give appropriate credit, provide a link to the license, and indicate if changes were made.
More articles you may like
Grandmothers: Innovation Through Tradition
Grandmother Project – Change through Culture (GMP) is an organisation dedicated to documenting the role of grandmothers and demonstrating the effectiveness of grandmother-inclusive strategies in improving the health and well-being of women, children, and adolescents. GMP’s groundbreaking work challenges conventional wisdom to transform community-based interventions in Africa and beyond, harnessing a powerful but often overlooked resource: the wisdom and influence of grandmothers.
Dr Robert Larkin | Cultivating Change to Improve Soil Health and Increase Potato Yield
Environmental quality and food production are facing the pressing challenges of climate change and global population growth. Dr Robert Larkin from the United States Department of Agriculture-Agricultural Research Service (USDA-ARS) and a team of plant scientists developed and tested a range of crop management systems to help overcome these compounding challenges. Their work is improving soil health and increasing the yield of potato crops, contributing to the future food security of nations.
Professor Giorgio Buttazzo | Artificial Intelligence and a Crossroads for Humanity
Where do we stand with artificial intelligence? Might machines take over our jobs? Can machines become conscious? Might we be harmed by robots? What is the future of humanity? Professor Giorgio Buttazzo of Scuola Superiore Sant’Anna is an expert in artificial intelligence and neural networks. In a recent publication, he provides considered insights into some of the most pressing questions surrounding artificial intelligence and humanity.
Dr Ralf Adam | New Technologies Shaping the Future of Oral Hygiene
Understanding the efficiency of various toothbrush technologies is essential for achieving optimal oral health. Dr Ralf Adam, who leads a dedicated team at Procter & Gamble in Germany, is keen to investigate the complexities of these technologies. His team have provided new insights into the best toothbrush types for plaque removal and the maintenance of gum health. By highlighting the importance of informed oral care decisions and ongoing investigations, this vital research works towards ensuring everyone can achieve a brighter, healthier smile.