The Highest Energy Li-ion Battery: Unlocking the Potential of the Silicon Anode and Nickel-rich NMC Cathode
Over the past decade, lithium-ion batteries have become essential to the portable electronics industry, and more recently have been championed as the transportation power source of the future. However, if electric vehicles are to gain widespread commercial success, modern lithium-ion batteries need to be cost effective, energy dense and long-lasting. Dr Daniela Molina Piper, Dr Tyler Evans, Dr Se-Hee Lee, and their team at SiILion Inc. have been completely rethinking the fundamental building blocks of these typical batteries, in order to develop an elegant solution to a significant modern problem.
A lithium-ion battery essentially consists of three components: two electrodes, and an electrolyte solution between them. The basic principle is that lithium ions contained within the electrolyte move from the positive electrode (cathode) to the negative electrode (anode) when the battery is charging, and back again when discharging. Almost all commercial lithium-ion batteries use an anode made primarily of graphite, long regarded as the most efficient material in its ability accept and release lithium ions during battery charging and discharging. The effective capacity of the battery is determined by the number of lithium ions that can reversibly travel between the anode and cathode material throughout battery operation.
The success of the lithium-ion battery is due to its many advantages over other contemporary batteries. Lithium-ion batteries have an almost negligible ‘memory effect’ (also known as the ‘lazy battery effect’) – a phenomenon that causes the battery to lose its ability to store charge (or its ‘capacitance’) with repeating recharges over time. They also have a large ‘energy density’, meaning that they have a high energy yield per unit volume of battery material. Additionally, they have low ‘self-discharge’ – a phenomenon whereby the stored charge in a battery becomes reduced, even when no device is connected to the electrodes.
To significantly reduce our carbon emissions, and thus mitigate the most disastrous consequences of climate change, replacing our gasoline-powered cars with battery-powered ones is an essential step. The lightweight nature of lithium-ion batteries, as opposed to a hefty lead-acid battery, make them an attractive option for this application. Indeed, most electric vehicles currently in development rely on a lithium-ion battery as their power source. However, the main problems that are incurred come down to battery capacity and longevity – the battery simply not having enough energy to power long journey distances. In order to be fully adopted as a viable transportation solution, the agreed estimate is that batteries need a gravimetric energy density of over 350 Watt-hours per kilogram (Wh/kg). Best efforts of current lithium-ion batteries fall far short of this, below 300 Wh/kg.

‘SiILion can integrate a wide range of silicon materials into its anode, to adapt to performance needs, and this also allows the SiILion cell design to realise the improvements that will arise due to new material introduction into the lithium-ion industry’ – Dr Molina Piper
A Change for the Better
Over the past 25 years, incremental design improvements have allowed lithium-ion batteries to increase their energy density by around 5–6% each year, but since their inception, the basic materials used in these batteries have remained unaltered. This slow improvement in performance is underwhelming, and the team at SiILion believes that this adherence to the same stale electrode materials is holding back the growth of the industry. A drastic break with the status quo in lithium-ion materials is needed if the exciting future adaptations predicted for lithium-ion batteries are to be achieved anytime soon.
Silicon anodes could be a suitable candidate to kick off this paradigm shift in lithium-ion batteries. In theory, silicon is an ideal replacement for graphite because of its low working potential versus lithium and high specific capacity, which is nearly 10 times higher than the most modern graphite anodes. As such, considerable research has been undertaken with the goal of creating a lithium-ion battery with a functioning silicon anode. However, in practice, a silicon anode has several drawbacks, particularly with its propensity to expand when the battery is charged.
The volume expansion of graphite anodes in most commercialised batteries is between 10–13%, while silicon’s expansion can be almost up to 300%. This expansion problem causes massive structural damage in the battery and compromises the fragile interface between the solid silicon electrode and the liquid electrolyte (the ‘solid-electrolyte interphase’, or SEI). Most modern research focuses on modifying the silicon material in a complicated way to accommodate more lithium ions without such severe expansion. The drawback of these modifications, however, is that they require intricate and costly processing methods, making this approach less appealing in the manufacture of commercial batteries. As Dr Evans of SiILion Inc. explains, ‘most of the published work around silicon anodes focuses on complex material modifications that ultimately will introduce manufacturing processes which are very difficult to scale’. A simple, scalable solution to the silicon anode is one key to higher performing batteries.
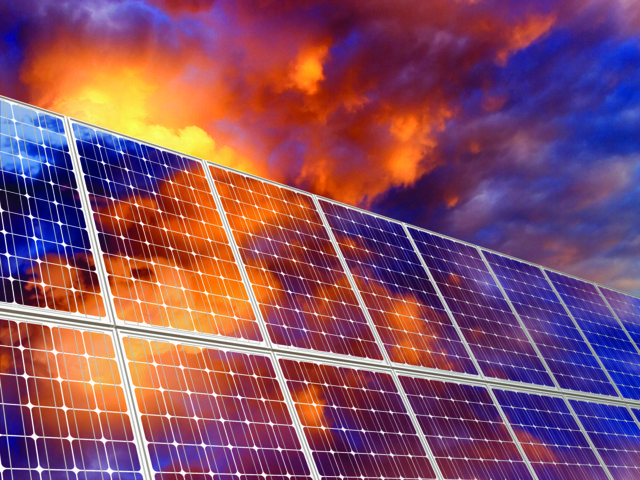
‘Most of the published work around silicon anodes focuses on complex material modifications that ultimately will introduce manufacturing processes which are very difficult to scale’ – Dr Evans
But What About the Cathode?
By improving the anode’s performance, you can increase a battery’s maximum energy output by 20–30%. However, in order to unlock the true potential of the battery, the cathode needs to be similarly enhanced. In terms of the cost of producing lithium-ion batteries, the cathode accounts for 30% of the total expense – more than twice that of the anode. Most conventional lithium-ion batteries use expensive and toxic cathodes, containing large amounts of cobalt, limiting their widespread application to electric vehicles.
Thus, cathodes containing large proportions of nickel are being explored as alternatives. Unfortunately, they are also problematic in the sense that they can be unstable at high temperatures and their structure may not be adequately robust. So, similar to the silicon anode, modern research is focusing on expensive and elaborate modifications to these nickel-based cathodes, which again will limit their commercial viability.
A Revolutionary Approach from SiILion Inc.
Dr Molina Piper, Dr Evans, Dr Lee, and their team at SiILion Inc. see the potential of these next-generation electrode materials, but also believe that the complex modification of the cathode and anode is not practical if these materials are to be applied to commercial battery systems. They claim that this method of combining next-generation materials with old-generation electrode and electrolyte designs, leads to obvious incompatibility issues. Because of this, the materials are increasingly over-engineered, while very little practical progress is made.
The team at SiILion is beginning to rewrite the rulebook for lithium-ion battery design. Not only do the cathode and anode materials need to be next-generation, the entire battery also needs revamping. They are shifting their focus to auxiliary battery materials, building a support system for the modern silicon and nickel-rich electrodes using unique electrolyte compositions and electrode binders, while maintaining the advantage of decades of manufacturing expertise by premising their designs on compatibility with existing lithium-ion battery manufacturing methods. As Dr Molina Piper explains, ‘enabling the next-generation electrode materials will mean enabling a next-generation lithium-ion system design’. Moreover, the strategy for attaining next-generation performance must be commercially viable. SiILion’s cell technology, through utilisation of lower cost materials and manufacturing compatibility, will be 30% less costly ($/kWh) than state-of-the-art lithium-ion cells. By approaching the problem from the view of the battery cell system, SiILion achieves its breakthrough energy density and performance.
Promising Results
With this philosophy in mind, the team at SiILion has undertaken the task of redesigning the old lithium-ion battery system, and their approach has already revealed some impressive achievements. SiILion has shown that when integrated into their unique system, state-of-the-art ‘nickel-rich’ cathodes and silicon anodes demonstrate a much-improved structural stability and safety, even at high temperatures. Dr Molina Piper also says that ‘SiILion has created the first viable 80% (by weight) silicon lithium-ion battery anode, capable of integration into standard electrode manufacturing processes’.
In fact, every silicon material that has been implemented into the SiILion system has shown an improvement in performance. ‘SiILion has worked with over two dozen types of silicon active materials (from over one dozen vendors), all showing marked improvement in capacity retention and coulombic efficiency when used in SiILion’s anode systems, regardless of the size or shape of silicon particles’ says Dr Evans. Of course, the team has its preferred material candidates, based on material stability and availability at scale. This ability to turn so many commercially available silicon materials into effective anodes could send shockwaves through the industry, making the current, over-engineered silicon anodes look overpriced by comparison.
The exact details of SiILion’s battery design remain confidential, but the problematic expansion effects that plague modern silicon anodes appear to have been overcome. As Dr Evans stated, the anodes display a minimal decrease in capacity after hundreds of charging and discharging cycles, and exhibit high ‘coulombic efficiencies’ – meaning that the charge is reversibly and effectively transferred through the system. This efficiency has been tested and proven with a variety of electrolyte materials, including coveted non-flammable electrolytes, which also shows the flexibility of the SiILion system.
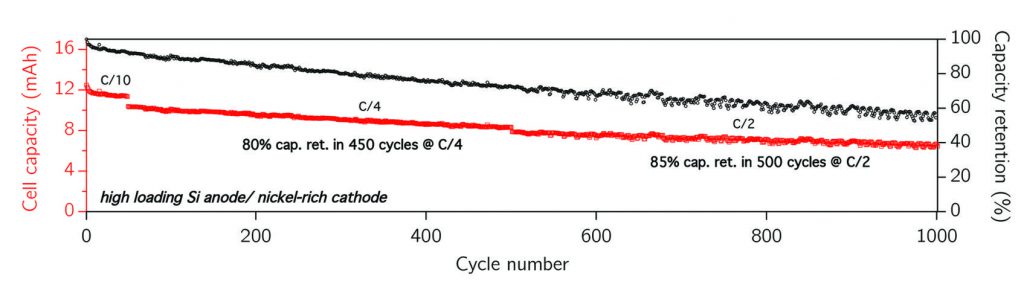
Figure highlighting SiILion’s cycling capability
Potential Applications
The real strength of SiILion’s battery system is its simplicity and its ability to be customised. The SiILion anode design can be employed with conventional electrolyte materials and a range of cathode materials, or can be employed with non-flammable electrolytes and next-generation, high-energy cathode materials. Crude, low-cost silicon materials, such as large particle ‘micron’ silicon materials can be incorporated, or more exotic anode active materials, such as silicon nano-wires or nano-featured silicon/carbon composites, could be employed when the system requires higher power with faster charging capabilities. SiILion’s major focus lies on pairing its anode designs with ionic liquid electrolyte materials that can be used for applications that need the highest energy density with an emphasis on the utmost degree of safety.
The team at SiILion particularly stresses the high emphasis they put on safety when designing their next-generation lithium-ion batteries. As anyone who is aware of the Samsung Galaxy Note 7 fiasco can attest, malfunctions involving lithium-ion cells have the potential to be catastrophic. If a faulty battery was scaled-up to the sizes required for electric vehicles, the consequences of malfunctioning would be even greater. With this in mind, SiILion’s use of a non-flammable electrolyte aims to eliminate the stigma that is associated with upscaling lithium-ion batteries to electric vehicle proportions.
In addition to the system’s versatility to accommodate the needs of various applications, the technology was specifically designed by SiILion so that it could be readily integrated into the existing infrastructure that is currently used to mass-produce lithium-ion batteries. This drastically reduces any costs that would be incurred for updating the current hardware, and makes the prospect of using this system commercially all the more attractive.

Figure highlighting SiILion’s scaling capability
What the Future Holds for SiILion
‘The SiILion team members have become experts in cell design around high loading silicon anodes, and this has proven very valuable,’ Dr Molina Piper states. ‘SiILion can integrate a wide range of silicon materials into its anode, to adapt to performance needs, and this also allows the SiILion cell design to realise the improvements that will arise due to new material introduction into the lithium-ion industry.’
So, building on their experience and expertise, SiILion’s next endeavour will be to optimise the material properties in their system, and then scale up the technology to truly demonstrate its value for the needs of electric vehicles and other applications. The company is now manufacturing 2.5 Ah prototypes, capable of achieving >300 Wh/kg, a pre-requisite step to ensuring that SiILion’s technology is inserted successfully into the markets. SiILion has also worked with its suppliers and manufacturing partners to validate its claims of lower cost, projecting a 25–35% cost saving, on a $/kWh basis, relative to current technologies.
Along the way to enabling vehicle electrification, SiILion is targeting the application of its technology in unmanned vehicles, specialty applications, and consumer electronics, with its current generation of prototypes designed to meet the requirements of these markets. With its business development efforts led by acting-CEO Mr Ed Williams, SiILion’s first generation prototype technology is under evaluation or requested for evaluation by numerous lithium-ion manufacturers and end-users active in its target markets. Ultimately, through development projects already underway and in the pipeline, SiILion is targeting a battery prototype that is predicted to deliver roughly 390 Wh/kg – far greater than any commercial lithium-ion battery currently available, and tantalisingly close to the energy density stated as optimal for electric vehicle applications.
Meet the researchers
Dr Daniela Molina Piper
SiILion Inc.
Broomfield, Colorado
USA
Dr Daniela Molina Piper leads SiILion’s early-stage operations as President and Chief Operating Officer. This includes fundraising, staffing, facilities establishment, day-to-day operations, contracts and project management, prototype development and design, and R&D. She obtained her PhD in Mechanical Engineering from the University of Colorado, Boulder, with her graduate research focused on silicon anodes for next-generation lithium-ion batteries, research that became part of SiILion’s core technology. Dr Molina Piper brings a unique blend of industry, academic, and National Laboratory experience to her role at SiILion.
CONTACT
E: dani.molinapiper@siilion.com
T: (+1) 484 241 1645
W: http://www.siilion.com/
Dr Tyler Evans
SiILion, Inc.
Broomfield, Colorado
USA
Dr Tyler Evans leads early-stage operations for SiILion as Chief Technology Officer. Responsibilities include fundraising, staffing, facilities establishment, day-to-day operations, contracts and project management, prototype development and design, and R&D. Dr Evans obtained his PhD in Mechanical Engineering from the University of Colorado, Boulder, and is a co-inventor of the SiILion technologies. Dr Evans’ research expertise focuses on enabling next-generation lithium-ion electrode materials by using advanced electrolytes.
CONTACT
E: tyler.evans@siilion.com
T: +1 303 905 7368
W: http://www.siilion.com/
KEY COLLABORATORS
Professor Se-Hee Lee, University of Colorado Boulder (co-founder)
Battery Innovation Center
Polaris Battery Laboratories
FUNDING
Angel Seed Fund
U.S. Department of Energy: SBIR Phase I, SBIR Phase II
U.S. National Science Foundation: SBIR Phase I
U.S. Department of the Navy: SBIR Phase I
Colorado Office of Economic Development and International Trade (CO OEDIT): AI ESCR grant
Asia-based automotive OEM: prototyping fund
REFERENCES
T Evans, DM Piper, H Sun, T Porcelli, SC Kim, SS Han, YS Choi, C Tian, D Nordlund, MM Doeff, C Ban, S-J Choi, KH Oh, S-H Lee, In Situ Engineering of the Electrode-Electrode Interface for Stabilized Overlithiated Cathodes, Advanced Materials, 2017, 29, 1604549.
DM Piper, T Evans, K Leung, T Watkins, J Olsen, SC Kim, SS Han, V Bhat, KH Oh, DA Buttry, S-H Lee, Stable Silicon-Ionic Liquid Interface for Next-Generation Lithium-Ion Batteries, Nature Communications, 2015, 6, 6230.