The National Institute of General Medical Sciences
As one of the 27 Centers, Institutes and Offices within the National Institutes of Health (NIH), the National Institute of General Medical Sciences (NIGMS) supports basic research that increases our understanding of biological processes, laying the foundation for finding new ways to predict, prevent, diagnose and treat disease. Often referred to as NIH’s ‘training Institute’, NIGMS’s second aim is to foster the best trained, most innovative and productive biomedical research workforce possible.
With a budget of $2.5 billion USD per year, NIGMS funds scientists at universities, medical schools, hospitals and research institutions throughout the US. Scientists funded by NIGMS investigate how living systems work at a range of levels, from molecules and cells to tissues, whole organisms and populations. Key areas of NIGMS investment include cell biology, biophysics, genetics, developmental biology, biochemistry, organic chemistry, biomedical technology, bioinformatics and computational biology. The Institute also supports research in certain clinical areas, primarily those that affect multiple organ systems, such as pharmacology, anaesthesiology, wound healing, physical trauma, burn injury and sepsis.
In this exclusive interview, we have had the pleasure of speaking with Jon R. Lorsch, Director of NIGMS, who describes the Institute’s unique and varied approaches to supporting basic biomedical research. He also elaborates on several cutting-edge techniques that are advancing our understanding of human health and disease, and shares his hopes for the future of NIGMS and fundamental biomedical research.
‘No matter how counter-intuitive it may seem, basic research has proven over and over to be the lifeline of practical advances in medicine’ – Arthur Kornberg, 1959 Nobel Laureate in Physiology or Medicine
You have had quite a successful and varied academic career – what motivated you to join NIGMS in 2013 as Director?
While at Johns Hopkins I steadily took on more leadership and managerial roles. I noticed that by serving in these roles, one can have a very big (and hopefully, positive) impact on a large number of people. In fact, it became clear that I could have a bigger impact on biomedical research – and potentially on society – through scientific leadership, management and administration, than I could by just focusing on my own lab research. I decided that helping to steer the scientific ship is the most important way I can spend my time.
NIGMS focuses on supporting investigator-initiated research, whereby the individual scientists identify the most important questions and develop the strategies needed to answer them. How successful is this approach?
History indicates that letting scientists ‘follow their noses’ is the most productive path to medical and technological breakthroughs. Targeted projects focused on a particular application can be vitally important, for instance, the development of a vaccine for an emerging viral disease. But investigator-initiated research, which involves a combination of curiosity, expertise, creativity and serendipity, has repeatedly proven to yield discoveries that are the most broadly applicable and transformative. By emphasising investigator-initiated fundamental research, NIGMS is unleashing the creativity and energy of scientists across the country.
Biomedical research is a dynamic enterprise that creates opportunities to explore new ideas. We are committed to giving investigators the freedom and flexibility to pursue emerging research questions that can lead to novel scientific insights.
We also encourage researchers to collaborate with each other, especially across disciplines, to form research teams. Only by combining the approaches and expertise of multiple scientific fields can we fully understand the complexities of living systems.
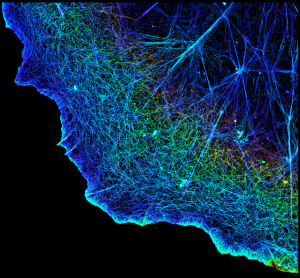
Researchers captured this detailed view of cellular scaffolding using a ground-breaking technique called stochastic optical reconstruction microscopy (STORM). The 2014 Nobel Prize in Chemistry honoured STORM and related microscopy techniques that are revolutionizing cellular imaging. CREDIT: Xiaowei Zhuang, Howard Hughes Medical Institute, Harvard University, and Nature Publishing Group.
Please describe the NIGMS Technology Development Program, and explain how it differs from previous programs supporting technology development in biomedical fields. What types of projects do you hope to see initiated under this new program?
To continue making discoveries, scientists need cutting-edge tools. To that end, NIGMS supports the development of, and widespread access to, high-quality technologies, such as laboratory instruments and techniques, computational technologies, chemical reagents, biological repositories and database resources. Such new technologies enable and expedite scientific advances that, in turn, drive the development of additional technologies.
Even more significant, novel technologies open unexplored fields of knowledge by allowing new types of questions to be asked. Think about what the discovery of microscopes allowed us to do – see life at the cellular level, diagnose diseases, and now, even manipulate single molecules and cells. Or another example – the ability to obtain complete genome sequences made it possible to compare genomes across individuals, revealing the genetic basis of a wide variety of human diseases. None of these advances would have occurred without the enabling technologies that made them possible.
We recently launched two initiatives to kick-start the development of new research tools and technologies. The initiatives are called Exploratory Research for Technology Development and Focused Technology Research and Development. Unlike the way technology development has historically been supported by NIH, these initiatives focus entirely on developing tools, not on applying them. Although every funded project must be motivated by an unmet need or new opportunity in biomedical research, the researchers are free to focus completely on the technical aspects of designing innovative new tools, rather than optimising existing tools to answer specific biomedical questions or explore particular organisms, systems or diseases.
NIGMS funds many scientists who develop and apply cryo-electron microscopy in their research. Tell us a bit about this powerful technique and its potential to increase our understanding of life at the molecular level.
Figuring out a molecule’s detailed, three-dimensional structure – the position and orientation of every atom inside it – is one of the ways researchers can learn about the molecule’s role in the body. This knowledge can then help pave the way for a wealth of medical advances, including the prevention and treatment of diseases.
In past decades, the most popular technique for studying the structure of molecules was X-ray crystallography, a technique unsurpassed in its ability to reveal intricate, atomic-level details, even for large molecular complexes. But this technique also has serious drawbacks – most notably, that it requires scientists to grow crystals of the molecules they are studying.
Growing crystals of most biological molecules is difficult, and occasionally impossible. Coaxing crystals to form requires putting the molecules in an unnatural environment and, at times, lopping off parts of them. Even then, the crystals may never form, or if they do, the data collected after taking these extreme measures might not accurately represent the structure of the molecule in its native environment.
Cryo-electron microscopy (cryo-EM) is emerging as complementary – and, in some cases, a promising alternative – to X-ray crystallography. In the past, cryo-EM was only able to reveal the fuzzy outline of a molecule, while crystallography provided exquisite internal details. Now, the visualisation power of cryo-EM is beginning to rival that of X-ray crystallography, thanks to recent advances in the cameras used to collect cryo-EM data and the computational methods used to process the data. Because it doesn’t require sample crystallisation, cryo-EM has the added advantage of enabling scientists to view specimens frozen from their natural or near-natural state. The power of cryo-EM improved so quickly and dramatically that the journal Nature Methods named it the Method of the Year in 2015.
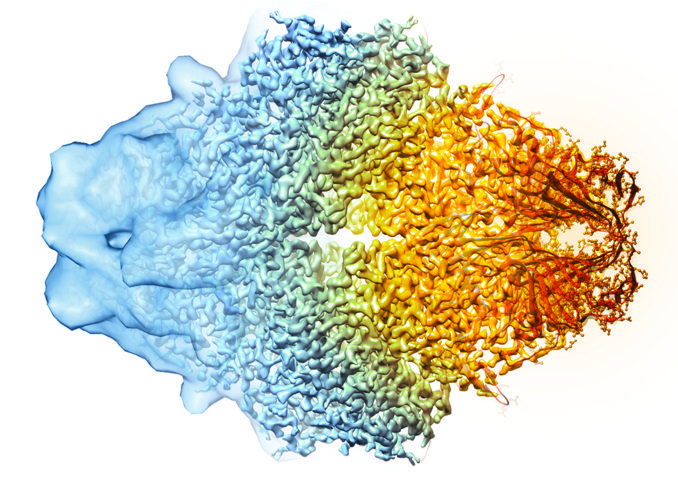
This image, showing a single protein molecule, is a montage. It was created to demonstrate how dramatically cryo-EM has improved in recent years. In the past, cryo-EM was only able to obtain a blobby approximation of a molecule’s shape, like that shown on the far left. Now, the technique yields exquisitely detailed images in which individual atoms are nearly visible (far right). CREDIT: Veronica Falconieri, Subramaniam Lab, National Cancer Institute.
‘Basic technological innovation can open new realms of scientific possibility’ – Francis Collins, Director, National Institutes of Health
Cryo-EM works on samples that are cryogenically frozen at liquid nitrogen temperatures. The flash-freeze is so quick that water molecules do not have time to form ice crystals, which could disrupt the normal arrangement of molecules. Samples prepared this way are very stable, and many images can be collected from them, allowing researchers to repeatedly refine their data, removing artefacts and creating even sharper images than ever before.
Some scientists are using cryo-EM to create three-dimensional models, or maps, of molecular complexes. To make these models, scientists use sophisticated software programs to stitch together thousands of two-dimensional images taken at slightly different angles. The resulting highresolution three-dimensional models are already yielding new information about the molecular underpinnings of health and disease. For example, recent advances towards a possible HIV vaccine depended on information from cryo-EM studies about how antibodies recognise the virus.
The Institute also focuses on dataintensive and data-driven biomedical research. Describe the importance of improving researchers’ ability to take full advantage of the ever-growing volume of complex biological data.
Over the past decade, the life sciences have been deluged with data, much of it stemming from genome-based technologies. We now have vast quantities of information about molecular networks, cellular processes and diseases. We have collections of databases, knowledge bases and repositories. The challenge is to figure out how data from multiple sources can be shared, analysed, integrated and interpreted in a seamless and synergistic way.
To get a feel for the scale of the problem, take genomic repositories as an example. There are more than a dozen of them, each focusing on a particular research organism – mouse, fruit fly, E. coli, etc. These repositories were created by and for the relevant community of scientists and evolved organically over many years. As an unfortunate result, the various resources are organised differently, maintained separately, and not integrated with one another.
There are also databases about biomolecules: protein structures go in one place, chemical structures in another. Other resources catalogue the DNA, RNA, proteins and small molecules involved in metabolism, signal transduction, immune system function, disease progression and many other processes. But there is no free and easy flow of information between these resources.
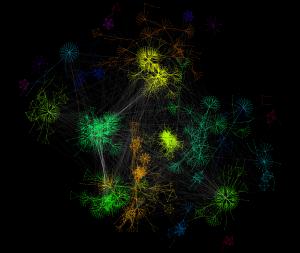
This diagram represents some of the thousands of known physical and genetic interactions inside a yeast cell. Many of the same interactions occur in humans. Computational tools, such as the software that created this diagram, enable the analysis of large amounts of data, such as all the proteins involved in cell movement, or all the genes activated in response to a toxic chemical, or all the genetic differences between two closely related species. CREDIT: Keiichiro Ono and Trey Ideker, University of California, San Diego.
Scientists and organisations worldwide are working to get all these biomedically relevant resources to ‘talk’ to each other. They even came up with an acronym for the goal – FAIR, for Findable, Accessible, Interoperable, Reusable. The idea behind the FAIR Principles is to not only benefit human researchers doing data-driven projects but also enable machines – computers – to find and analyse data from multiple resources in an automated way.
For our part, NIGMS fosters a stable, robust and FAIR data ecosystem by supporting new technologies in biomedical computing, informatics and data sciences. NIGMS-funded researchers are forging new applications that will help bridge the gap between the current model of data production and a newer model of data-derived knowledge.
NIGMS also supports a variety of projects that use research organisms. Give a few examples of how this type of research has advanced the understanding of human health and disease.
Much of what we know about biology was learned through research on organisms such as bacteria, fruit flies and mice. These studies taught us how cells grow and divide, how inheritance works, how organisms store and use energy, how certain parts of the brain function, how diseases develop, and what drives behaviours such as eating and sleeping. These lessons have led to new methods for addressing health and disease in humans.
At the most basic level, all life is virtually identical. All organisms rely on the same systems, structures and substances to live. That’s why research on non-human organisms can shed light on human biology. Regardless of where on the tree of life a creature resides, it uses similar genes, proteins, hormones and other molecules to sense and respond to the environment; digest, store and utilise food; build, transport and dispose of materials; and grow, develop, reproduce, age and die. As a result, a well-designed experiment in a tiny roundworm could shed light on a biological process in people.
In general, research organisms are small, relatively easy and inexpensive to work with, and widely available to scientists. They might also have characteristics that are desirable for certain research projects. For example, zebrafish grow quickly and have see-through embryos, making them ideal for studying how organs develop. Mice that have specific diseases or genetic defects are available by mail order and have been used to investigate cancer, diabetes, infectious diseases, neurodegenerative diseases, autoimmune disorders, birth defects, drug addiction and hundreds of other disorders.
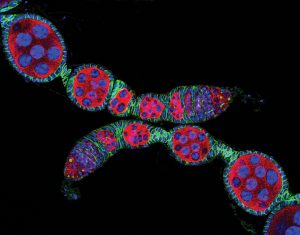
The fruit fly Drosophila melanogaster is one of the first widely used research organisms. It has been studied for more than a century. Research on Drosophila has shed light on many aspects of human biology, including learning, memory and neurodegenerative diseases. Shown here are ovarioles, the tubes in which egg cells form. CREDIT: Daniel Kirilly in the Ting Xie Lab, Stowers Institute for Medical Research, and the 2004 Olympus BioScapes Competition.
Studies in fruit flies and tiny worms revealed key aspects of how fertilised eggs develop into complex organisms. This research also led to the unanticipated discovery that genetically controlled cell death plays a critical role in cancer and other diseases. As another example, scientists studied yeast to sort out the orderly sequence of events that cells follow to duplicate their contents and divide into two, a process called the cell cycle. This information impacts millions of people, as many anti-cancer drugs interfere with the cell cycle. Furthermore, research in nematode worms has yielded important information about ageing. This research is very difficult to do in people and other organisms that have long life spans.
Studies in research organisms have also produced many powerful scientific tools, including CRISPR, RNA interference, DNA chips and genome-wide scanning methods. These tools are now being used in human health studies worldwide.
Researchers are constantly seeking and discovering new research organisms. A relatively new example is the bobtail squid – a strawberry-sized creature that lives in knee-deep waters around Hawaii. These miniature squid have a symbiotic relationship with bioluminescent bacteria known as Vibrio fischeri. The bacteria inhabit a special light organ on the squid’s underside. There they are fed a nutrient-rich solution in exchange for providing light that a bobtail squid can tune to match its surroundings. Cloaked in ambient light, the squid’s silhouette and shadow essentially disappear, making it invisible to predators. Juvenile bobtail squid actively encourage V. fischeri bacteria to colonise their light organs. If no colonisation occurs, the squid cannot reach sexual maturity.
Humans also have symbiotic relationships with bacteria and other microorganisms. These creatures live everywhere in and on us: our guts, skin, noses, mouths, lungs. They are essential for proper nutrition and may be involved in normal immune system development, neurological function and more. They may also play roles in the development of diabetes and other diseases.
Scientists have begun studying the symbiosis between bobtail squid and V. fischeri as a model to better understand the complex relationship between humans and our resident microorganisms and how this dynamic partnership influences our health and development.
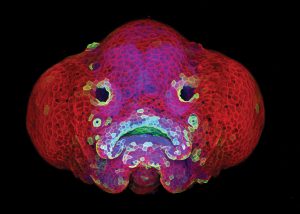
Zebrafish are a relatively new research organism. The zebrafish’s rapid growth and see-through embryo make it ideal for scientists studying how organs develop. In this six-day-old zebrafish larva, what look like eyes will become nostrils. The bulges on either side of the fish’s head will become eyes. This image won a 2016 BioArt award from the Federation of American Societies for Experimental Biology. CREDIT: Oscar Ruiz
and George Eisenhoffer, University of Texas MD Anderson
Cancer Center, Houston.
Finally, what do you see as the biggest challenges facing basic biomedical research over the next decade? Tell us about the Institute’s plans to tackle these challenges.
Over the past decades, basic biomedical research has flourished, leading to a deeper, more sophisticated understanding of living systems. As we build on these advances, we aim to deploy our resources in the most efficient ways possible. We also need to include a wide variety of scientists so we can benefit from the full spectrum of perspectives on biomedical questions. To accomplish these goals, we need to re-optimise the scientific enterprise.
Specifically, I’d like to see NIGMS:
• Create more efficient and sustainable funding mechanisms for investigators at different stages of their careers.
• Modernise graduate school education to take advantage of the latest educational methods and equip our future scientists with 21st-century skills.
• Ensure rigor and reproducibility in research.
• Increase diversity in the workforce and in the institutions, geographic regions, scientific topics and approaches we support.
We have covered all these topics in the NIGMS Feedback Loop blog, and will continue to do so, but here are a number of highlights:
Funding mechanisms: Historically, most NIGMS research grants funded individual projects that had specific goals defined at the beginning of a four- to five-year project period. Sometimes, during a project, observations and insights reveal exciting new research questions or unexplored areas. Under the current system, it can be difficult for investigators to pursue new directions using funds that were allocated for a specific purpose. In addition, scientists who want to launch new projects must apply for additional grants. Any time spent writing and reviewing grant applications means less time for conducting research.
To address these issues, NIGMS initiated a new, five-year funding mechanism called the Maximizing Investigators’ Research Award (MIRA). Rather than requiring scientists to focus on specific projects, this award provides support for any research in their laboratories that falls within the NIGMS mission. MIRA is designed to produce a more stable, flexible and efficient research environment. We hope it will allow scientists to be more productive and more innovative – to take scientific risks and to pursue important, new scientific questions that arise during the course of their research. We have a companion MIRA program for early-stage investigators that aims to help young scientists overcome hurdles in becoming independent scientists.
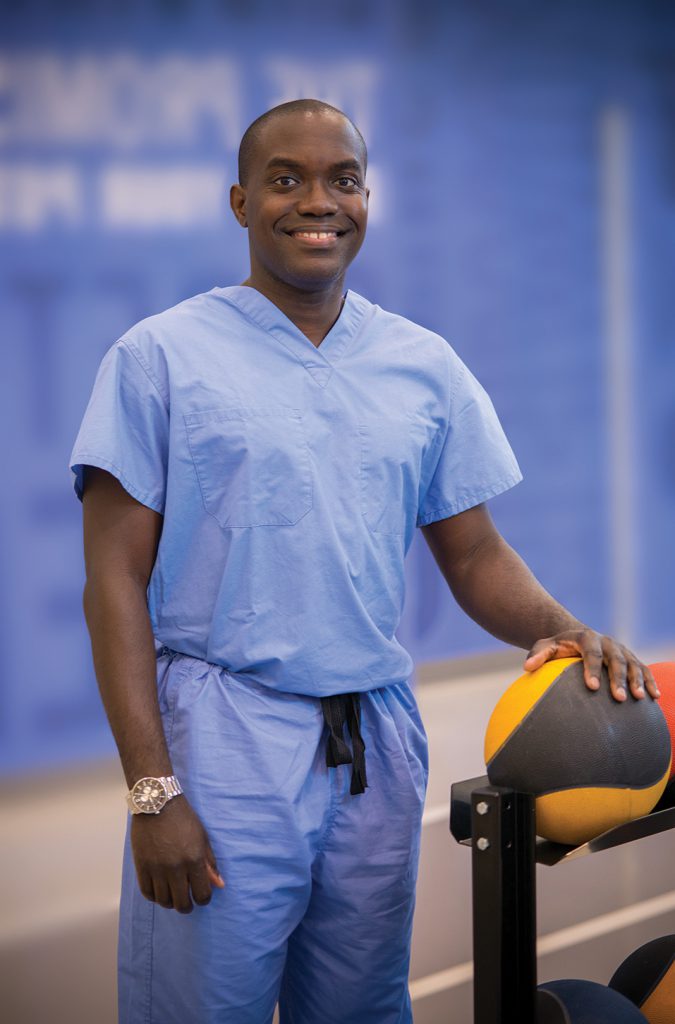
Alfred Atanda, Jr., is a paediatric orthopaedic surgeon and researcher at Nemours/Alfred I. duPont Hospital for Children in Wilmington, Delaware. He receives grant support through an Institutional Development Award (IDeA) that provides mentoring and professional development opportunities to investigators in the early stages of their careers. CREDIT: Cynthia Brodoway, Nemours/ Alfred I. duPont Hospital for Children.
Modernising graduate school education: Training the next generation of biomedical researchers is fundamental to the NIGMS mission. Toward that goal, NIGMS is actively supporting efforts to catalyse the modernisation of biomedical graduate education. We have undertaken several initiatives to stimulate this process, such as hosting a symposium to showcase innovations in biomedical graduate education, career development and skills development. Future training issues we want to address include: enabling institutions to innovate to find optimal models for training scientists in the 21st century, identifying best practices for educators and mentors as they design and implement new education and training models, and tracking student outcomes and evaluating program results.
Rigor and reproducibility: To accurately reveal the complexities of living systems, biomedical research must be rigorous. Experiments should be robust and unbiased, and they should produce results that are high quality, reliable and reproducible. In recent years, a number of publications have suggested that the rigor and reproducibility of biomedical experiments are eroding. NIH is leading the effort to address this concern and has been joined by the research community, scientific publishers, universities, industry and professional organisations.
NIGMS is addressing these issues through several initiatives, including co-hosting a trans-NIH workshop on reproducibility in cell culture studies; providing extra funds to more than a dozen training grants to teach graduate students how to design high-quality experiments; and supporting, along with nine other NIH components, grants for training modules to enhance data reproducibility, then publishing the products of these grants in an online clearinghouse.
Diversity and inclusion: Achieving diversity in the workforce is also a key priority for the Institute. The biomedical workforce has not kept pace with changes in the nation’s demographics. As a result, the field is not benefiting from the creativity, energy, skill sets and viewpoints of many within the US populace. To better leverage the rich diversity of thinking and experiences within our country, NIGMS offers several programs designed to develop talented people from underrepresented populations.
In addition, NIGMS aims to broaden the geographic distribution of NIH funding. Through the Institutional Development Award (IDeA) program, NIGMS boosts research capacity through faculty and student development and building research infrastructure in states that historically have not received high levels of NIH funding.
The overarching goal for all of NIH is to advance medical science and improve human health. My hope is that, through our support of investigator-initiated research, effective training programs and new technologies, NIGMS will play an important role in reaching that goal.