Professor Peter Santschi – Cleaning Up a Catastrophe
Professor Peter Santschi and his team at Texas A&M University are dedicated to investigating the consequences of the release of radioactive substances into the environment. Incorrect storage of nuclear waste or power plant accidents can cause radioactive material to spread away from the source in the environment, contaminating the surrounding ecosystem with a radioactive potency that can persist for centuries. Professor Santschi and his colleagues perform important work by studying the mobility of these radioactive elements within the environment, providing necessary information for assessing the environmental and human risk posed by these contaminants.
Recently, power generated from nuclear energy has undergone something of a renaissance due to growing concerns about greenhouse gas emissions. Countries such as India and China have pledged to build dozens more nuclear power plants in the upcoming decade. It’s easy to see why, when you consider that a single gram of uranium-235 releases almost 300,000 times the energy of an equivalent coal source, with the added benefit of producing negligible amounts of greenhouse gas emissions. Whilst a fuel like uranium-235 isn’t technically classified as a renewable energy source, it has been predicted that with future advances in reactor efficiency, especially with regards to recycling the waste, current reserves could potentially power the entire globe for 100,000 years.
Fundamentally, a nuclear reactor works by bombarding a heavy, radioactive atom such as uranium with neutrons in order to make it more unstable. This instability causes the radioactive nucleus to split into smaller atoms, emitting further neutrons in the process. This then sets off a chain reaction of uranium-235 atoms being split, and dividing it into smaller more stable atoms. Known as nuclear fission, this process produces an enormous amount of energy in the form of heat, which is then utilised to drive steam turbines in order to generate electricity with a high degree of efficiency.
While there are many advantages, working with nuclear energy is well-known for being a double-edged sword. The waste produced in the nuclear fission chain reaction is a major drawback of this power source. If not handled correctly, nuclear waste can be incredibly harmful to humans, and if released into the environment, it can have devastating consequences to any surrounding plant and animal life. It therefore has to be stored responsibly, with every effort made to ensure that there are minimal leakages for thousands of years. However, despite all the precautions taken, leakages of radioactive waste into the environment have happened multiple times throughout history.
Just Another Day at the Office
Professor Santschi and his team carry out their work at some of the world’s most irradiated areas. Most of these sites became contaminated over 50 years ago, with the initial discovery of nuclear reactions, and the production of nuclear weapons and their testing. This sparked the arms race and the Cold War. In the past, less was known about the immense dangers of nuclear waste, or the contamination in the surrounding environments at some of these production sites. Two of these facilities, where Professor Santschi and colleagues conduct their research, are the Savannah River Site in South Carolina and the Hanford Site in Washington State. These sites were formally used by the Department of Energy to make nuclear materials for defence purposes, as opposed to energy purposes.
This leaked radioactive waste has the potential to then spread throughout the environment in a number of ways. As an example, rainwater can wash the radionuclides (radioactive atoms) down through the soil and into the underlying aquifer where it can be transported to any number of aquatic environments. At the Hanford Site alone, there are hundreds of acres of surface aquifer contaminated with radioactivity that are presently undergoing remediation.
Professor Santschi and his team also study samples from the site of the infamous Fukushima Daiichi Nuclear Power Plant in Japan. When in 2011, an earthquake-induced tsunami struck the plant, the wave’s impact destroyed its power source and backup generators. The reactor units were then improperly cooled down and the reactor core went into meltdown. This catastrophe caused the release of massive amounts of radioactive substances into the surrounding atmosphere and into the Pacific Ocean. Thankfully, disasters like this are uncommon, but when they do occur, the sheer volume of radioactive material released can have a devastating impact on the surrounding environment.
Releasing Radioiodine
A common waste product of the nuclear fission reaction are two forms of radioactive iodine, known as iodine-129 and iodine-131. With a difference of only two neutrons, iodine-129 and iodine-131 have radically different properties. The isotope iodine-131 is produced much more readily in fission reactions, but is much more short-lived, with a half-life of only eight days. This means that for a given sample of iodine-131, half of the atoms in the sample will have decomposed into non-radioactive atoms after just 8 days. In contrast, iodine-129 is less common in nuclear fallout, but as a beta emitter with a half-life of 16 million years, measuring it is more challenging. While these forms of radioiodine occur naturally in tiny quantities, the majority present in the world comes from human activity, due to accidental leakage or nuclear disasters.
Iodine is required by the human body, with almost all of it being stored in the thyroid gland, where hormones for controlling growth and metabolism are produced. If the body were to be exposed to large amounts of radioiodine, it would be collected and stored here in the same way. This has been the case for workers of the Chernobyl and Fukushima power plants, where a nuclear disaster exposed them to massive amounts of radioactive particles. Tragically, the radioiodine that was absorbed and concentrated in their thyroid, bombarded the surrounding tissue with high doses of radiation, has the potential of leading to cancer.
Although all forms of radioiodine are dangerous, the dangers of iodine-131 are not as stark as those of iodine-129. Because of its short lifetime, iodine-131 doesn’t pose as significant a threat to humans and the environment. Ironically, it can actually be used to treat thyroid cancers, as it releases radiation that can kill cancer cells, and then swiftly decays into the stable and harmless element xenon-131. Iodine-129 is much more problematic because of its long-lasting potency, and its tendency to bond with other molecules.
Ground-Breaking Research
Professor Santschi and his team have been studying the effects of this radioiodine interacting with the environment for over 10 years. He realised that when radioiodine or any other radionuclide is released, reactions will occur between these hazardous substances and the neighbouring natural organic matter. Professor Santschi explains that his research ‘asks the question: what organic molecules carry radionuclides in the environment?’ He goes on to say that ‘answering this question requires one to bridge the gap between environmental radiochemistry and environmental organic chemistry, which normally are two separate scientific disciplines with each asking their own questions, and requiring very different training and knowledge.’
Radionuclides that are exposed to the environment go through several complicated reactions with surrounding matter. When exposed to aquatic environments, radioiodine can exist in multiple chemical forms (oxidation states) and can bind strongly to natural organic matter, making environmental remediation challenging. Standard models for predicting how nuclear waste propagates through the environment often assume that radionuclides remain in the same oxidation state and fail to take into account interactions with natural organic matter.
Professor Santschi and his colleagues developed a brand-new approach to determine the amount of iodine present in the environment. They used a technique known as Gas Chromatography/Mass Spectrometry (GC-MS), as well as Fourier Transform Ion Cyclotron Resonance Mass Spectrometry (FT-ICR-MS) and Nuclear Magnetic Resonance (NMR) at their collaborator’s facilities, to give much more detailed insight into what forms of iodine were present in the soil. Previous techniques could only measure iodine at very high concentrations that would never be found naturally, so the real breakthrough of this technique is that it allows the various iodine species, and isotopes, to be detected at concentrations that are environmentally relevant.
Complex Reactions
When iodine-129 is created as a by-product of nuclear power and released to the environment, it can become negatively charged by accommodating an extra electron. This form of iodine ion is known as ‘iodide’, and most conventional models only account for this form when attempting to calculate the dispersion of radioiodine. As iodine can exist in seven different oxidation states, Professor Santschi and his team realised that the reality is much more complicated. These differently charged iodine ions behave in completely different ways and will either flow more easily or less easily into the environment depending on the state.
There are many ways in which radioiodine can be altered when exposed to the environment. If the extra electron of iodide becomes lost to a surrounding substance, another iodide will be able to bond with it to produce the diatomic molecule I2. I2 is how elemental iodine exists under standard room temperature and pressure conditions; however, the bond is not particularly strong, so further reactions can occur if I2 is in the presence of oxygen. Most commonly, a threefold negatively charged species, IO3–, called ‘iodate’ is expected to form. Radioiodine can also bind in numerous ways to the carbon in natural organic matter. All of these reactions make radioactive iodine more likely to bond to natural organic matter compounds, creating stable organic iodine molecules. The group found clear evidence that this organo-iodine formation is mediated by enzymatic reactions and microbial activity. The stability of all of these molecules changes the way the iodine would usually propagate, making it more difficult to extract and clean up.
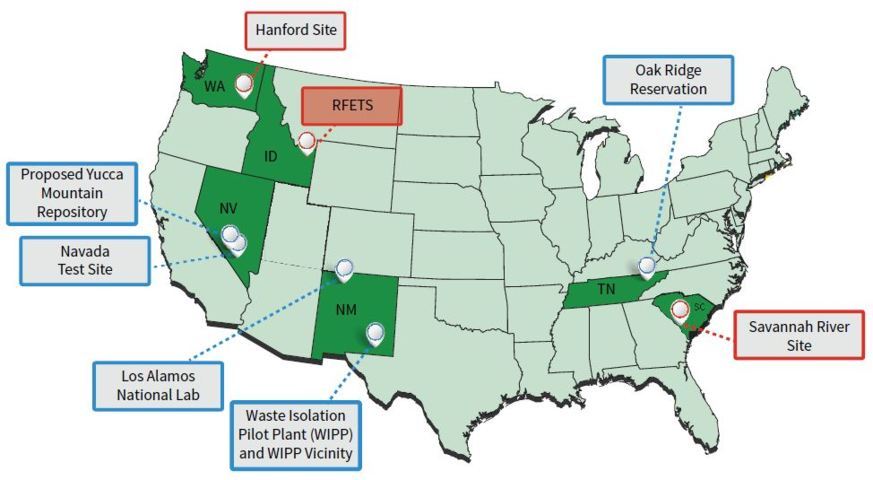
USA nuclear waste sites
Professor Santschi’s group also found clear evidence that another radionuclide, plutonium – also a potentially toxic radionuclide – is strongly bound to specific natural organic matter compounds in surface soils. However, unlike iodine, which is directly bound to aromatic compounds similar to how it occurs in our body, plutonium was found in the company of strongly iron-binding compounds called ‘hydroxamate siderophores’ in chemically separated plutonium enriched fractions. Hydroxamate siderophores are among the strongest binding agents for plutonium, with some of them binding even stronger to plutonium than to iron. These compounds are naturally produced to mobilise iron from soils to make iron (which is normally insoluble) available to microorganisms and plants. However, these compounds were unequivocally found in the macromolecular and particulate fraction. The team concluded that it is highly likely that plutonium is bound to those siderophores, catching a ride on the ‘iron wheel’.
For both elements (iodine and plutonium), their strong binding to naturally produced organic macromolecules under environmentally relevant concentrations has shown unexpected behaviour with respect to their mobilisation and immobilisation potential. Professor Santschi’s group’s low-level approach simulating ambient behaviour is complementary to synchrotron radiation techniques that are commonly applied by radiochemists – approaches that work only at higher concentrations. However, the team showed the importance of working at ambient environmental concentrations, where their behaviour can be different from that expected at higher concentrations.
Environmental Impact
The significance of studying these less-mobile radionuclide-containing molecules is that they can potentially pose a much greater health risk to humans. The standard form of radioactive iodide, for example, can spread easily throughout the environment, making it more diluted and less of a significant health risk. However, the forms of iodine that are more likely to remain in soil pose different risks. While it is beneficial that these forms are less mobile, and therefore can be monitored more effectively and potentially recovered, less mobile molecules remain in the soil for a significant amount of time, and may be taken up by plant matter and incorporated into their structure. Once taken up by plants, herbivorous livestock can consume this radioactive fodder, converting it into muscle tissue, which would be carcinogenic to humans if eaten as meat. Alternatively, radioiodine that reacts in aquatic environments can be taken up by fish in a similar way, which would then become equally harmful if consumed.
The importance of this work can have impacts in all areas of the environment. Professor Santschi explains that principle of these techniques could help not only environmental remediation of existing contaminated sites, but also provide information that can be used to estimate risk posed by accidental release, such as Fukushima, and thus create information that is being used to minimise risk posed by long term disposal of waste.
Meet the researcher
Professor Peter H. Santschi
Department of Marine Sciences
Texas A&M
Galveston, TX
USA
Professor Peter Santschi obtained his PhD in Chemistry in 1975 from the University of Bern in Switzerland. Since then, he has worked as a researcher at various prestigious institutions, such as Columbia University, the Swiss Institute for Water Resources and Water Pollution Control and the National Taiwan University, to name just a few. Professor Santschi is currently a Professor in the Department of Marine Sciences at Texas A&M University. His research interests include a broad range of topics in Marine and Environmental Chemistry, including the role of natural nanoparticles in the biogeochemical cycling of trace substances, tracer applications using radioactive and stable isotopes, relationships between trace elements and natural organic matter, and the importance of exopolymeric substances for trace element binding and removal from natural waters. Over the course of his career, Professor Santschi has been the recipient of many awards and honours, including the Distinguished Achievement Awards in Research, as well as Graduate Student Mentoring from Texas A&M, and he is an elected Geochemical Fellow of the Geochemical Society, the European Association of Geochemistry and Fellow of the American Geophysical Union.
CONTACT
E: santschi@tamug.edu
T: (+1) 409 740 4476
W: http://loer.tamug.edu/people/Santschi/index.html
KEY COLLABORATORS
Chen Xu, Saijin Zhang, Kathleen A. Schwehr, Russell Grandbois, Texas A&M University
Daniel I. Kaplan, Savannah River National Laboratory
Chris M. Yeager, Los Alamos National Laboratory
Patrick M. Hatcher, Old Dominion University
Yuko Sugiyama, University of Hyogo, Japan
Nobuhito Ohte, Kyoto University, Japan
Nobuhide Fujitake, Kobe University, Japan
Shigeyoshi Otosaka, Japan Atomic Energy Agency, Japan
Seigo Amachi, Chiba University, Japan
FUNDING
Department of Energy, Office of Science, Subsurface Biogeochemistry Research (SBR) program
National Science Foundation, Division of Ocean Sciences, Chemical Oceanography Program
REFERENCES
PH Santschi, C Xu, S Zhang, KA Schwehr, P Lin, CM Yeager, DI Kaplan, Recent Advances in the Detection of Specific Natural Organic Compounds as Carriers for Radionuclides in Soil and Water Environments, with Examples of Radioiodine and Plutonium, Journal of Environmental Radioactivity, 2017, 171, 226–233.
C Xu, S Zhang, Y Sugiyama, N Ohte, YF Ho, N Fujitake, DI Kaplan, CM Yeager, K Schwehr, PH Santschi, Role of Natural Organic Matter on Iodine and 239,240Pu Distribution and Mobility in Environmental Samples from the Northwestern Fukushima Prefecture, Japan, Journal of Environmental Radioactivity, 2016, 153, 156–166.
C Xu, S Zhang, DI Kaplan, YF Ho, KA Schwehr, KA Roberts, H Chen, N DiDonato, M Athon, PG Hatcher, PH Santschi, Evidence for Hydroxamate Siderophores and Other N-Containing Organic Compounds Controlling 239,240Pu Immobilization and Remobilization in a Wetland Sediment, Environmental Science & Technology, 2015, 49, 11458–11467.
DI Kaplan, ME Denham, S Zhang, C Yeager, C Xu, KA Schwehr, HP Li, YF Ho, D Wellman, PH Santschi, Radioiodine Biogeochemistry and Prevalence in Groundwater, Critical Reviews in Environmental Science and Technology, 2014, 40, 2287–2335.
C Xu, H Chen, Y Sugiyama, S Zhang, HP Li, YF Ho, CY Chuang, KA Schwehr, DI Kaplan, C Yeager, KA Roberts, PG Hatcher, PH Santschi, Novel Molecular-Level Evidence of Iodine Binding to Natural Organic Matter from Fourier Transform Ion Cyclotron Resonance Mass Spectrometry, Science of the Total Environment, 2013, 449, 244-252.