Dr Gerhard Haerendel – Exploring the Vibrant Dynamics of Near-Earth Space
The region of space in which Earth’s magnetic field interacts with flowing charged particles is home to a rich array of physical processes – but studying them is no easy task. Through a career spanning over 50 years, Dr Gerhard Haerendel at the Max Planck Institute for extraterrestrial physics has carried out world-leading research into these processes. His discoveries have now led to ground-breaking insights in the field of plasma physics – including explanations of striking arcs in the aurora, the discovery of characteristic prominences on the Sun’s surface, and analysis of artificial comets seeded directly into space.
Near-Earth Space
To the naked eye, the region of space just above Earth’s atmosphere may appear to be empty and static. In reality, however, this region is an ever-changing landscape of dynamic interactions between charged particles, which are dominated by the influence of Earth’s magnetic field. At its lower end, the region of space where these processes occur is bordered by the ionosphere: the part of Earth’s atmosphere that contains particles ionised by the Sun’s radiation. At its upper end, it is responsible for deflecting streams of charged particles originating from the Sun – named the solar wind – protecting Earth’s surface from this damaging radiation.
Interspersed throughout near-Earth space is a thin plasma composed of negatively charged electrons, and positively charged ions. As it moves, this fluid generates its own magnetic field, which itself interacts with Earth’s magnetic field, as well as those in the ionosphere. This interplay between charges and fields creates a deeply complex web of interactions which, for researchers, is notoriously difficult to study. In order to disentangle these dynamics, researchers have developed a wide array of sophisticated techniques over past decades.
Seeding Clouds in Space
When Dr Gerhard Haerendel first started out in his career, simulation-based techniques were not nearly powerful enough to capture these processes. In order to understand them, researchers instead attempted to study them through elaborate experiments in space. In 1969, Dr Haerendel became the head of one such group at the MPI for extraterrestrial physics, which fired rockets, and later satellites, directly into near-Earth space. At their destinations, these vessels released, or ‘seeded’ clouds of barium directly into the surrounding natural plasma.
‘For 25 years I was involved in experiments with sounding rockets and later satellites for the purpose of seeding artificially produced plasmas in space and study their dynamics and interactions with the natural plasma environment,’ explains Dr Haerendel.
Once seeded, the neutral atoms in the clouds were ionised by the Sun’s radiation, causing them to emit photons with frequencies characteristic to barium. By picking up these signals using sensors on the ground, Dr Haerendel and his colleagues could study how their seeded cloud evolved over time, and how it interacted with its surrounding environment. By the early 1980s, these experiments had provided researchers with a far greater understanding of the processes that play out above Earth’s atmosphere. Overall, his team’s efforts culminated in a monumental experiment, which has never been attempted since: placing artificial comets directly into space.
Creating Artificial Comets
Comets are small, icy bodies, which are perhaps best characterised by their two striking tails. Flowing out from a single ‘head’, one of these tails is made of ice and dust particles, while the other is made of plasma. Furthermore, both tails always point away from the Sun, due to the combined influence of the solar wind, and pressure imparted by the Sun’s radiation. Between December 1984 and July 1985, Dr Haerendel’s team used NASA’s AMPTE satellites to provide unprecedented insights into how comets’ tails form, and how they interact with plasma in the solar wind.
To do this, the researchers released two canisters of barium from the satellite, and then exploded them simultaneously. This produced a rapidly expanding cloud, which engulfed the German spacecraft built by Max Planck Institute in just a fraction of a second. Dr Haerendel and his colleagues then saw that that the barium gas was swept away as it was ionised by the Sun’s ultraviolet radiation, forming a visible tail, distinct from the head, which travelled at speeds of tens of kilometres per second.
To analyse this behaviour, the physicists measured the density and motions of ions and electrons in different parts of the evolving cloud. This allowed them to explore fundamental characteristics of the interaction between the cloud, and plasma in the solar wind. They concluded that their artificial comet’s behaviour was comparable to Earth’s ‘bow shock’ – an obstacle to the solar wind, which forms as the magnetosphere interacts with the solar wind. Overall, this ground-breaking experiment provided critical new information about the interactions taking place in near-Earth space. In the coming decades, theoretical techniques would also improve significantly, and would soon become a key element of Dr Haerendel’s research.
Arcs in the Aurora
Above the Earth’s poles, the inward-curving shape of its magnetic field enables the solar wind to interact with the ionosphere. The results of these interactions are the colourful swirls of light that can be seen in polar skies, known as the aurora. One particularly striking feature of the aurora is the emergence of widely extending luminous arcs generated by the impact of energetic electrons – whose source has long remained a mystery. Leading on from experiments in which his team directly seeded barium clouds into these arcs, Dr Haerendel discovered that they are a result of ‘fracturing’ magnetic fields in the ionosphere.
This theory states that under growing shear forces exerted by the upper magnetosphere, opposite-facing frictional forces in the ionosphere cause stress to build in its magnetic field, which can be released suddenly. This occurs as fractures appear in the field, where electrical currents, trapped in 2D ‘sheets’, develop field-aligned voltages. Here, auroral arcs will form as the plasma and field undergo rapid stress releasing motions. For the first time, this discovery allowed Dr Haerendel’s team to characterise the physical properties of these structures by parameters including their widths, as well as their time-varying motions.
Reconnections Between Field Lines
In parallel research, Dr Haerendel and his team studied how magnetic fields in near-Earth space can undergo ‘reconnection’ – a process in which field line shapes are suddenly rearranged due to external forces. Just as processes like tearing or joining paper involve far more energy than simply bending it, this process can oversee the dramatic release of magnetic energy, in the form of kinetic and thermal energy. In the environment of near-Earth space, this process can have profound effects on the behaviours of plasmas and magnetic fields, but its influence still needs further exploration.
Dr Haerendel has recognised that within an area of low plasma density, situated at a few thousand kilometres above the Earth’s surface, fractures in the magnetic field are enabled by field-aligned voltages, which are responsible for the acceleration of particles in the aurora. The fracturing causes the magnetic fields in this region to become temporarily disconnected from fields anchored in the ionosphere and replaced by new connections. A dramatic release of energy is involved in this process, which becomes invested in the acceleration.
Related Dynamics on the Solar Surface
Dr Haerendel’s research has not been limited to near-Earth space. Elsewhere, he has explored how similar principles can be applied to magnetic fields close to the Sun. In particular, he was the first to explain the cause of downflowing plasma in ‘prominences’. First observed by instruments aboard the Hinode satellite in 2008, these structures form mysteriously thin, vertically oriented threads above the Sun’s surface, with steady down-flowing velocities of 20 kilometres per second.
Through his analysis, Dr Haerendel determined that these flows are made up of plasma squeezing through vertical field lines under the influence of gravity, dragging magnetic fields with them. Eventually, these fields disconnect from that of the prominence as a whole, and reconnect with others – eventually forming smaller packets of plasma. Such behaviour is remarkably similar to how water breaks up into droplets when flowing over a mountain ridge.
Alongside this work, Dr Haerendel has studied magnetic reconnection associated with more familiar phenomena, known to astronomers as solar flares, which are closely related to dramatic eruptions of plasma from the Sun’s surface. Through this analysis, he reconsidered previous theories of the process to account for reconnection in the Sun’s magnetic field, which is continually being sheared due to subsurface motions. These calculations enabled Dr Haerendel to develop a new model that modifies, for the first time, the brightness, sizes, and flow speeds of ribbon-like features in the flares on account of field-parallel acceleration processes.
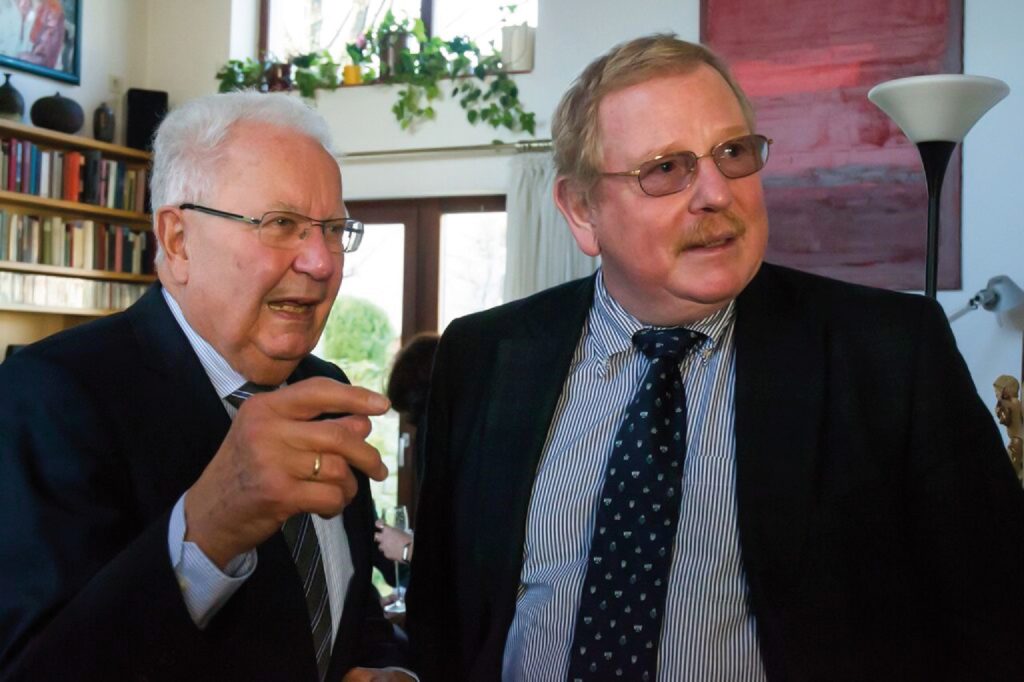
Dr Haerendel on his 80th birthday with Dr Reinhard Genzel
Suddenly Stopping Plasma
Back close to Earth, Dr Haerendel has considered the complex physics underlying flows in the aurora, as they interact with the field of plasma in the magnetosphere’s ‘tail’. Facing away from the Sun, this feature arises since the solar wind compresses Earth’s magnetic field on its daytime side, allowing it to extend far out into space on the night-time side – in a remarkably similar process to that observed in Dr Haerendel’s earlier comet seeding experiments. Within the strongly stretched field of the magnetic tail, large amounts of plasma – and subsequently, a large reservoir of energy – can be stored.
Through theoretical considerations, supported by independent lab-based experiments, Dr Haerendel became the first to show how a ‘stop layer’ forms at the interface between plasmas arriving from reconnection in the tail and the magnetic field, acting like an abrupt wall. From these results, he has shown how this mechanism causes the momentum and energy of plasma in the tail to be coupled directly to fields in the outer magnetosphere and from there to the ionosphere – in a direct reversal of the magnetic reconnection process.
A Successful Career of Research
While the interactions of multiple systems of plasma and their associated magnetic fields are notoriously difficult to model, Dr Haerendel’s ground-breaking research has significantly advanced researchers’ comprehension of the physical processes involved. By building on the new physical insights that he has pioneered throughout his career, one may hope to understand these processes in even more detail in the future. Aside from areas ranging from the vibrant behaviours of the aurora, to the ever-changing dynamics playing out on the Sun’s surface, his insights may have numerous applications within other astrophysical situations.
Reference
https://doi.org/10.33548/SCIENTIA593
Meet the researcher
Dr Gerhard Haerendel
Max Planck Institute for extraterrestrial physics
Garching
Germany
Dr Haerendel has over 50 years of experience in space research, since he began his PhD studies in Physics at the University of Munich. After his graduation in 1963, he worked at the Max Planck Institute for extraterrestrial physics, where he became Director of the Institute in 1972. Here, he was responsible for a series of plasma cloud experiments, which led to ground-breaking results in the fields of plasma and magnetospheric physics. Dr Haerendel held this position until retiring in 2000, and has since taken on numerous different roles – including the founding dean of the School of Engineering and Science at Jacobs University in Bremen, as well as being the co-founder of the first astronomical observatory on the island of Crete. Over the course of his successful career, he has been Vice President of the International Academy of Astronautics, President of the Committee on Space Research (COSPAR) and Chairman of the European Space Science Committee (ESSC).
CONTACT
E: hae@mpe.mpg.de
W: https://www.mpe.mpg.de/personnel/17794
KEY COLLABORATORS
Prof. Dr Reimar Lüst, founder of the Max Planck Institute for extraterrestrial Physics, subsequently president of the Max Planck Society, deceased
Dr Götz Paschmann, Max Planck Institute for extraterrestrial Physics, retired
Dr Erich Rieger, Max Planck Institute for extraterrestrial Physics, retired
Dr Arnoldo Valenzuela, Max Planck Institute for extraterrestrial Physics, retired
Prof. Dr Wolfgang Baumjohann, Institute for Space Research, Austrian Academy of Sciences
Prof. Dr Yannis Papamastorakis, University of Crete, retired
Dr Stephen Mende, Space Sciences Laboratory, Univ. of California, Berkeley
Dr Harald Frey, Space Sciences Laboratory, Univ. of California, Berkeley
FUNDING
Funding for this research was dominantly and continuously provided from the Max Planck Society.
Most of my space projects received additional funding from the Ministry of Research and Technology of the Federal Republic of Germany.
FURTHER READING
G Haerendel, Experiments with plasmas artificially injected into near-Earth space, Frontiers in Astronomy and Space Sciences, 2019, 6, 29.
Haerendel, G, Reconnection Mediated by Magnetic Fractures and the Solar Flare, The Astrophysical Journal, 2018, 855, 95.
G Haerendel, L Suttle, SV Lebedev, GF Swadling, JD Hare, GC Burdiak, SN Bland, JP Chittenden, N Kalmoni, A Frank, RA Smith, Stop layer: a flow braking mechanism in space and support from a lab experiment, Plasma Physics and Controlled Fusion, 2016, 58, 064001.
G Haerendel, TA Berger, droplet model of quiescent prominence downflows, The Astrophysical Journal, 2011, 731, 82.
G Haerendel, Magnetic fractures or reconnection of type II, Proceedings of the International Astronomical Union, 2010, 6, 56.
G Haerendel, Auroral arcs as sites of magnetic stress release, Journal of Geophysical Research: Space Physics, 2007, 112, A09214.
Want to republish our articles?
We encourage all formats of sharing and republishing of our articles. Whether you want to host on your website, publication or blog, we welcome this. Find out more
Creative Commons Licence
(CC BY 4.0)
This work is licensed under a Creative Commons Attribution 4.0 International License.
What does this mean?
Share: You can copy and redistribute the material in any medium or format
Adapt: You can change, and build upon the material for any purpose, even commercially.
Credit: You must give appropriate credit, provide a link to the license, and indicate if changes were made.
More articles you may like
Dr Timothy Beers | Mapping the Galaxy’s Stellar Populations Using Large Photometric and Astrometric Surveys
Astronomers often use spectroscopic (electromagnetic radiation) data and astrometric (motion and positional) data to develop working models describing our Galaxy. Dr Timothy Beers from the University of Notre Dame and his collaborators in Korea and China combined large photometric (visible light) surveys and astrometric data to create multidimensional maps of a large part of the Galaxy. By highlighting significant inhomogeneities in stellar-chemical compositions, motions, and spatial distributions, Dr Beers and his colleagues provide valuable insights into how we can advance our understanding of the formation and evolution of our Galaxy.
Dr Anushia Inthiran | Distance Learning: Impacts for Offshore Students Amid COVID-19
The COVID-19 pandemic significantly disrupted global education and necessitated a shift to online learning. Due to ongoing border closures, even after the pandemic eased, offshore students were prevented from attending their university in person long after their local peers, impacting their learning ability and future perspectives. Dr Anushia Inthiran from the University of Canterbury conducted a survey among a group of offshore students to understand the consequences of distance learning on their education.
Dr Sébastien Weber | PyMoDAQ: Navigating the Future of Data Acquisition
In an era where data is paramount, Dr Sébastien Weber and his team at CNRS, the French National Centre for Scientific Research, are changing the landscape for scientists and engineers with PyMoDAQ, an open-source data acquisition software. Their revolutionary tool stands out for its accessibility, versatility, and the thriving community it fosters.
Professor Gary Yohe | Navigating Climate Change: The Impactful Contributions of Gary Yohe
Professor Gary Yohe is a distinguished environmental economist whose work has been pivotal in shaping our understanding of climate change impacts, adaptation strategies, and policy frameworks. His interdisciplinary approach combines economics with environmental science, offering nuanced insights into global warming and its multifaceted impacts on natural and human systems. Professor Yohe equips us with the knowledge and strategies needed to navigate the complex and pressing challenges posed by climate change.