Dr Joe Huba – Plasma Bubbles after Sunset: Simulating Instabilities in the Ionosphere
Many kilometres above the Earth’s equatorial region, something strange occurs for several hours in the late evening: vast bubbles of plasma form in the upper atmosphere, which quickly rise upwards into space. Dr Joe Huba at Syntek Technologies in Virginia aims to gain a better understanding of this complex process, by recreating it through computer simulations. His team’s work is providing researchers with a more complete understanding of Earth’s atmosphere, and could also provide critical insights for satellite systems that communicate using radio waves, as well as global positioning systems.
Earth’s Ionosphere
If you look at a picture of Earth taken from space, the atmosphere will appear as a thin, fragile layer surrounding our home planet. Yet as we can clearly see by simply looking up at the sky, there is a diverse range of dynamic physical processes at play within the atmosphere, giving rise to effects including the weather we experience on Earth’s surface.
At different altitudes, variations in air density, temperature and wind speed can have significant influences over these processes, resulting in several distinctive layers, each with its own defining characteristics. Among these layers is a region named the ‘ionosphere’, which extends from 60 kilometres to over a thousand kilometres. This layer’s defining characteristic is that the atoms and molecules it contains are partially ionised by powerful ultraviolet radiation from the sun.
The neutral atmosphere, known as the thermosphere at ionospheric altitudes, plays an important role in the dynamics of the ionosphere. Thermospheric winds interact with the plasma and generate large-scale electric fields, which can transport the plasma in altitude and latitude.
Within the ionosphere, several smaller regions can also be found. Among them is a high concentration of plasma named the F-region, situated between 150 and 800 kilometres above sea level. As many physicists are well aware, the intricately complex motions of plasma particles can give rise to many interesting behaviours, making the F-region an especially interesting part of the atmosphere. In the ionosphere above Earth’s tropics, one particularly intriguing display begins to take place after sunset.
Instabilities in Immiscible Fluids
On smaller scales, the physics underlying this effect can be seen in the interaction between two immiscible fluids, such as oil and water. When the denser fluid is supported by the less dense fluid, an instability arises that creates a ‘bubble’ inside the denser fluid. This bubble accelerates and expands as it travels upwards into the denser fluid. This fluid instability is known as the Rayleigh-Taylor instability.
This complex scenario can be found in a wide range of situations in nature, from the clouds thrown up by volcanic eruptions, to cosmic explosions created by supernovae. To study them, researchers can construct computer simulations that recreate the properties of each fluid, enabling them to accurately reproduce the effects of instability.
In the lower latitudes surrounding Earth’s equator, a comparable effect emerges in the ionosphere’s F-region, which could be studied through similar simulations in principle. However, this situation is more complex than many cases studied previously – creating a unique set of challenges for Dr Joe Huba and his colleagues.
Equatorial Spread F
In the evening, after sunset, the ionosphere can be suddenly lifted to higher altitudes because of the electric field generated by the thermospheric wind. Under these circumstances, a sharp density gradient develops with the electron density increasing with altitude. The situation becomes similar to oil and water: a dense (heavy) plasma is supported by a less dense (light) plasma that becomes unstable. Irregularities can form large ‘bubbles’ of plasma, often tens of kilometres across.
These bubbles will then rise rapidly towards high altitudes, expanding and accelerating in the process. Afterwards, they can remain for several hours after sunset and sometimes past midnight. Now known as the ‘equatorial spread F’ (ESF), this phenomenon has a profound impact on the behaviour of the ionosphere surrounding the bubbles. In turn, it also has a significant effect on some of the radiation passing through the ionosphere – which cannot be ignored in the modern age of communication.
Unwanted Effects from Scintillation
For astronomers, an effect known as ‘scintillation’ is an ever-present challenge. Most commonly, it describes the random fluctuations experienced by radio waves as they pass through the streams of charged particles generated by the sun – known as the solar wind.
The effect is comparable to the twinkling we see when we look up at the stars at night. In this case, random turbulence in the atmosphere slightly diverts the paths of light emitted by stars, which we observe as twinkling. Similarly, radio waves can fluctuate as they pass through charged solar wind, causing inconvenient distortions of the signals emitted by distant astronomical objects due to effects including absorption, scattering, and frequency shifts.
Dr Huba’s team notes that the effect can become distinctly more noticeable for radio waves passing through the ionosphere at just the wrong time. As the plasma bubbles produced by ESF rise to high altitudes, they will create enormous disturbances in the rest of the ionosphere, causing the layer to become unstable to shorter wavelength irregularities (a few centimetres to hundreds of meters). When this happens, the resulting scintillation of radio signals can become so strong that it renders them unrecognisable by the time they reach Earth. Yet while the bubbles occur infrequently enough to not be a significant problem for radio astronomers, the case is different for systems that depend on highly accurate signal transmissions.
Disrupting Satellites
In order to operate effectively, many of the satellites we now rely upon in our everyday lives must continually hover over a single point on Earth’s surface. To do this, they occupy a highly specific position in space called ‘geostationary orbit’ – consisting of a ring situated above the Earth’s equator. Today, this ring is home to satellites used for applications including communication, navigation, and weather forecasting.
The positioning of geostationary orbit presents a problem: the radio waves that its satellites use to communicate with facilities on Earth must often pass through the equatorial F-region, where any scintillation can cause significant disruption to signal exchanges that require pinpoint precision.
As an example, global navigation systems are required to measure the exact times taken for radio waves to travel between different satellites in an array, and a single device on Earth. If any of these signals pass through a plasma bubble, this timing information could be completely disrupted, leading to potentially damaging inaccuracies in global positioning. Without any way to prepare for these fluctuations, the systems that many people depend upon can become far less reliable for several critical hours in the late evening.
Barriers to Accurate Models
To alleviate these problems, physicists need to build computer simulations that can model, and subsequently forecast, when ESF bubbles will occur – affording the operators of satellite systems critical time to prepare for them. Yet as is the case for many simulations involving plasma, these models are notoriously challenging to build, since they must account for the intricate webs of interactions between the charged particles they contain. For a situation as complex as ESF, bubble plasma evolution is particularly difficult to predict.
In the past, researchers have simplified the situation by only simulating a more easily manageable 2D ionosphere. Later advances in computing technology have more recently allowed for 3D simulations. However, all of these efforts have so far faced several shortcomings: they model a limited region of the ionosphere, usually a few degrees in longitude and tens of degrees in latitude, and neglect realistic thermospheric waves.
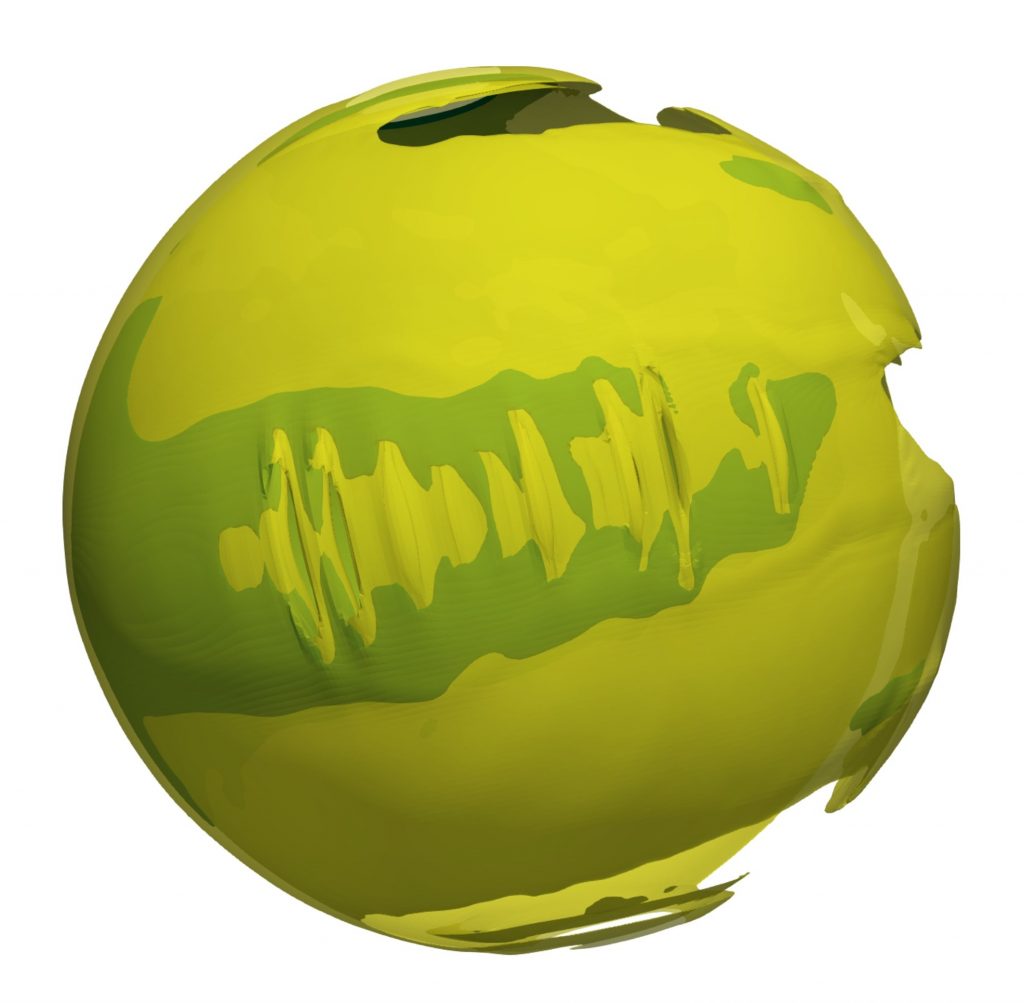
Global electron density (green represents denser regions and yellow indicates lower density). The corrugated structure in the density is a result of plasma bubbles at lower altitudes rising to higher altitudes.
Simulating Plasma Bubbles
Through their research, Dr Huba and his colleagues have accounted for this behaviour for the first time, by combining two global, first-principles atmospheric models: the whole atmosphere model WACCM-X and the ionosphere model SAMI3. Moreover, these models use very high grid resolutions, on the order of 70 kilometres. This allowed the team to recreate entire equatorial ionosphere driven by a realistic thermosphere model that includes atmospheric waves, such as gravity waves which can act as ‘seeds’ to trigger the instability.
To test the performance of their approach, they carried out the two simulation studies at two different times of year under different geophysical conditions: the ‘March case’ for low solar activity and the ‘July case’ for high solar activity.
Without any further input, Dr Huba and his colleagues found that their simulation self-consistently generated rising plasma bubbles. Furthermore, they found that in the March case – when the sun’s activity was lower – the bubbles penetrated to the upper reaches of the F-region, around 800 kilometres above sea level, where they persisted until after midnight. In contrast, this didn’t happen in the July simulation, during higher solar activity. Understanding why bubbles occur on some nights but not others is one of mysteries that these simulations hope to unravel.
The team compared the results to observations made by NASA’s GOLD mission, which continually monitors the ionosphere. Unexpectedly, the simulated behaviour was highly consistent with GOLD’s observations, proving that their approach resulted in the first reliable simulations of ESF plasma bubbles.
Informing Future Improvements
The reliability of the team’s simulation represents a significant step forward in our ability to understand the physics underlying ESF, and to prepare for the inconveniences created by rising plasma bubbles.
As we come to increasingly rely on satellites for communication and navigation, the outcomes of their research could soon lead to important new measures to safeguard and improve these systems. Through future research, they could also yield intriguing insights into the complex processes that play out above our heads, helping researchers in many different fields to understand more about the Earth’s atmosphere as a whole.
Reference
https://doi.org/10.33548/SCIENTIA526
Meet the researcher
Dr Joe Huba
Syntek Technologies
Fairfax, VA
USA
Dr Joe Huba completed his PhD in theoretical plasma physics at the University of Maryland in 1975. Following his graduation, he joined the Naval Research Laboratory as an NRC post-doc and eventually became a staff member. Between 1995 and 2018, he headed the Space Plasma Physics Section, part of the Beam Physics Branch at the Naval Research Laboratory. He now works as a Senior Research Scientist at Syntek Technologies, whose clients include the US Federal government. Dr Huba’s main research interests include boundaries and wave phenomena in plasma, processes involving Earth’s upper atmosphere and magnetic field, and approaches to modelling these systems. Over decades of research, he has been dedicated to writing advanced computer code to simulate these systems, which incorporate algorithms capable of simulating their sheer complexity.
CONTACT
E: jdhuba@syntek.org
E: jdhuba@gmail.com
KEY COLLABORATORS
Dr Hanli Liu, HAO/NCAR
Dr Jonathan Krall, Naval Research Laboratory
The original ionosphere model, SAMI3, was developed by Drs Huba and Joyce. Dr Joyce played a critical role in the code’s development but sadly passed away in 2011.
FUNDING
National Science Foundation (NSF)
National Aeronautics and Space Administration (NASA)
FURTHER READING
JD Huba, HL Liu, Global Modeling of Equatorial Spread F with SAMI3/WACCM‐X, Geophysical Research Letters, 2020, https://doi.org/10.1029/2020GL088258.
JD Huba, G Joyce, Global modeling of equatorial plasma bubbles, Geophysical research letters, 2010, 37, L17104.
HL Liu, BT Foster, ME Hagan, JM McInerney, A Maute, L Qian et al., Thermosphere extension of the Whole Atmosphere Community Climate Model, Journal of Geophysical Research, 2010, 115, A12302, https://doi.org/10.1029/2010JA015586.
JD Huba, G Joyce, J Krall, Three‐dimensional equatorial spread F modelling, Geophysical Research Letters, 2008, 35, L10102.
Want to republish our articles?
We encourage all formats of sharing and republishing of our articles. Whether you want to host on your website, publication or blog, we welcome this. Find out more
Creative Commons Licence
(CC BY 4.0)
This work is licensed under a Creative Commons Attribution 4.0 International License.
What does this mean?
Share: You can copy and redistribute the material in any medium or format
Adapt: You can change, and build upon the material for any purpose, even commercially.
Credit: You must give appropriate credit, provide a link to the license, and indicate if changes were made.
More articles you may like
Grandmothers: Innovation Through Tradition
Grandmother Project – Change through Culture (GMP) is an organisation dedicated to documenting the role of grandmothers and demonstrating the effectiveness of grandmother-inclusive strategies in improving the health and well-being of women, children, and adolescents. GMP’s groundbreaking work challenges conventional wisdom to transform community-based interventions in Africa and beyond, harnessing a powerful but often overlooked resource: the wisdom and influence of grandmothers.
Dr Robert Larkin | Cultivating Change to Improve Soil Health and Increase Potato Yield
Environmental quality and food production are facing the pressing challenges of climate change and global population growth. Dr Robert Larkin from the United States Department of Agriculture-Agricultural Research Service (USDA-ARS) and a team of plant scientists developed and tested a range of crop management systems to help overcome these compounding challenges. Their work is improving soil health and increasing the yield of potato crops, contributing to the future food security of nations.
Professor Giorgio Buttazzo | Artificial Intelligence and a Crossroads for Humanity
Where do we stand with artificial intelligence? Might machines take over our jobs? Can machines become conscious? Might we be harmed by robots? What is the future of humanity? Professor Giorgio Buttazzo of Scuola Superiore Sant’Anna is an expert in artificial intelligence and neural networks. In a recent publication, he provides considered insights into some of the most pressing questions surrounding artificial intelligence and humanity.
Dr Ralf Adam | New Technologies Shaping the Future of Oral Hygiene
Understanding the efficiency of various toothbrush technologies is essential for achieving optimal oral health. Dr Ralf Adam, who leads a dedicated team at Procter & Gamble in Germany, is keen to investigate the complexities of these technologies. His team have provided new insights into the best toothbrush types for plaque removal and the maintenance of gum health. By highlighting the importance of informed oral care decisions and ongoing investigations, this vital research works towards ensuring everyone can achieve a brighter, healthier smile.