Dr Rene Bellwied – Understanding the Nature of Matter through Quark Gluon Plasmas
In the extreme environment of the primordial universe, fundamental particles that are now tightly bound into larger groups were, for the briefest moment, free to wander individually. Using the latest particle accelerator facilities, researchers today can recreate fluids of these particles, named ‘Quark Gluon Plasmas’, through high-energy collisions between heavy ions. In his research, Dr Rene Bellwied at the University of Houston uses results from these experiments to explore the fascinating dynamics of the plasmas, and the products that emerge in their aftermath. His team’s findings are now shedding new light on the enigmatic nature of matter itself.
Quarks
Quarks are a fundamental part of much of the matter in the universe that we can observe. Although these particles can come in six possible ‘flavours’, most fall into just two such categories: these are the ‘up’ and ‘down’ quarks, which make up the protons and neutrons that surround and compose us.
For an absolutely vast majority of the universe’s history, virtually all quarks in the universe as far as we know have been bound to one or more additional quarks, and can never exist on their own. In protons and neutrons, for example, they exist in groups of three, which are held together by massless particles named ‘gluons’.
Over the past few decades, physicists have discovered a growing number of more exotic particles – composed of different types and combinations of quarks; and yet the rule that quarks must always be confined within larger particles continued to hold. Nonetheless, this wasn’t always the case: for the briefest moment following the Big Bang, temperatures were so colossally high that the rule could be temporarily broken. For roughly one trillionth of a nanosecond, quarks and gluons existed as individual particles within an enigmatic, ultra-hot fluid. Today, physicists call this elusive substance a ‘Quark Gluon Plasma’.
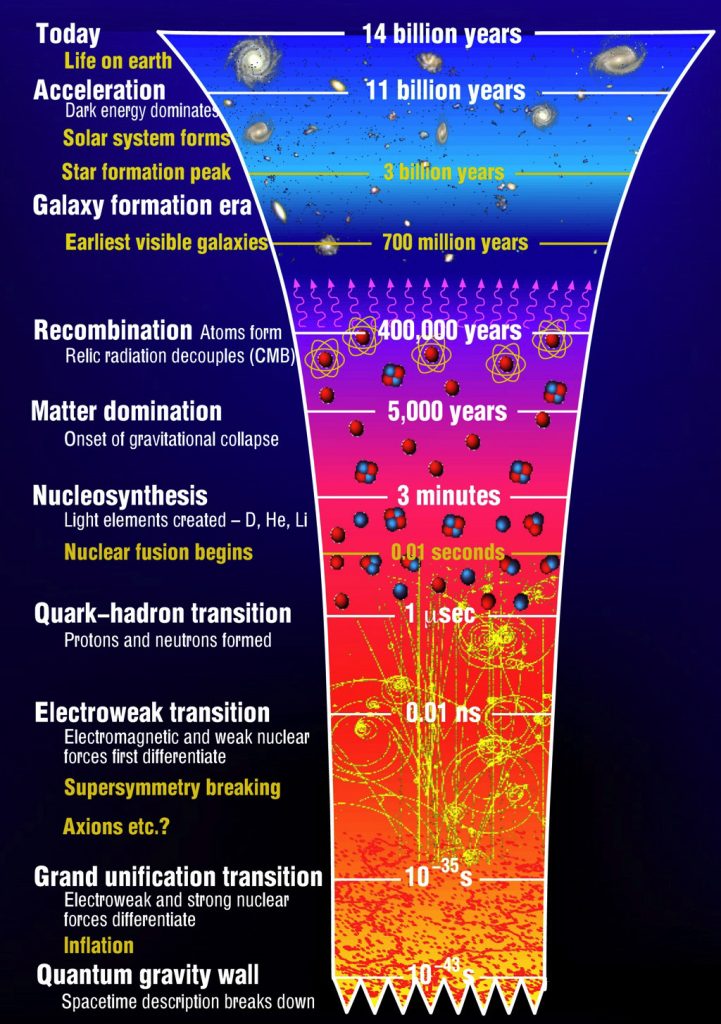
Evolution of the universe as a function
of time.
Recreating the Early Universe
The brief era where Quark Gluon Plasmas dominated the universe may now be in the distant past – but thanks to the latest generation of particle accelerators, researchers can now recreate this primordial environment in controlled lab conditions. At its core, the concept behind their experimental process is fairly simple: they must release as much energy as possible, within the smallest possible space. To do this, researchers can accelerate subatomic particles to an appreciable fraction of the speed of light, smash them together, and observe the particles that emerge in the aftermath.
To maximise the energy – and thereby the temperatures and densities of these collisions – physicists use ions of heavy, stable elements such as lead, which carry far more momentum than smaller particles when travelling at the same speed. Over the past two decades, their efforts have yielded promising results. ‘Through experiments at the Relativistic Heavy Ion Collider at Brookhaven National Laboratory on Long Island, and the Large Hadron Collider at CERN, Geneva, we have established that the matter at these high temperatures and densities can transition into a Quark Gluon Plasma,’ says Dr Rene Bellwied of the University of Houston.
Just like in the early universe, the fluid doesn’t stick around for long. As Quark Gluon Plasmas created in the aftermath of high-energy collisions cool down, they will rapidly ‘re-confine’ – with independent quarks and gluons grouping together to form new particles. Most often, this will result in new protons and neutrons – yet as Dr Bellwied and his colleagues have discovered, the re-confinement process can be highly varied. With the right approach, researchers can use their analysis of re-confinement to gain a deeper understanding of the nature of matter itself.
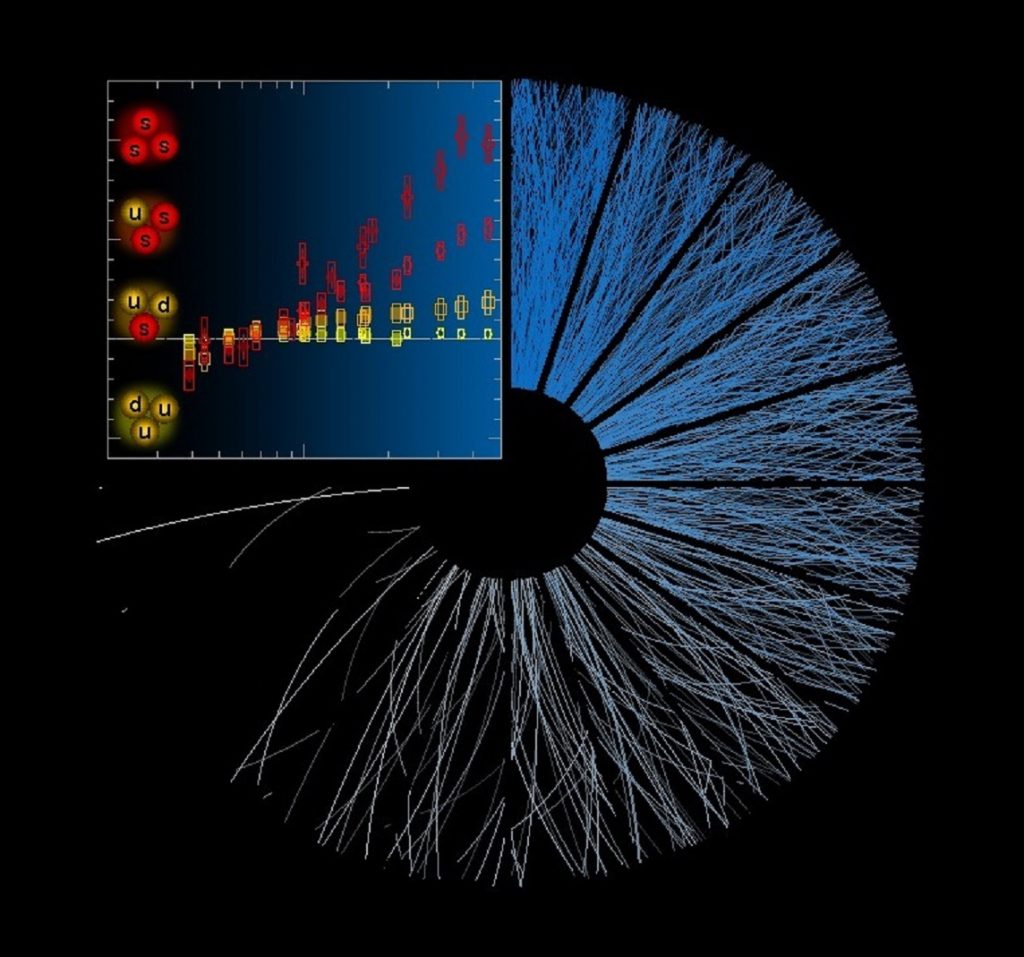
Enhancement of strange particles as a function of number of
strange quarks in the particles. Nature Phys., 13, 535.
New Experimental Opportunities
The ability to generate Quark Gluon Plasmas on demand has opened up a wide array of opportunities for particle physics experiments in recent years. As Dr Bellwied explains, two lines of research in this area are particularly important. ‘Firstly, we characterise the new short-lived state of matter and its properties to learn about the fundamentals of free quarks and gluons,’ he says. ‘Secondly, we attempt to understand the formation of matter and new forms of matter through studying the transition back out of the Quark Gluon Plasma.’
This first aspect of Quark Gluon Plasma research can perhaps yield the most immediate benefits to our understanding of particle physics. As fundamental particles, quarks and gluons are both key elements of the Standard Model, which describes the nature of all matter in the universe according to our current understanding. With the ability to study the physical properties of individual particles, free from the influence of others, researchers could improve the predictions of the Standard Model, and perhaps even expose some of its limitations.
In his research, Dr Bellwied focuses more closely on the second aspect he describes. By studying the Quark Gluon Plasma re-confinement process and the products of its aftermath in more detail, his team’s findings are providing new insights into aspects of the nature of matter that cannot be proved through more conventional experiments. Three specific areas of his research are particularly intriguing, including particles containing ‘strange’ quarks, the enigmatic contents of ultra-dense neutron stars, and the mysterious influence of quantum mechanics on plasma dynamics.
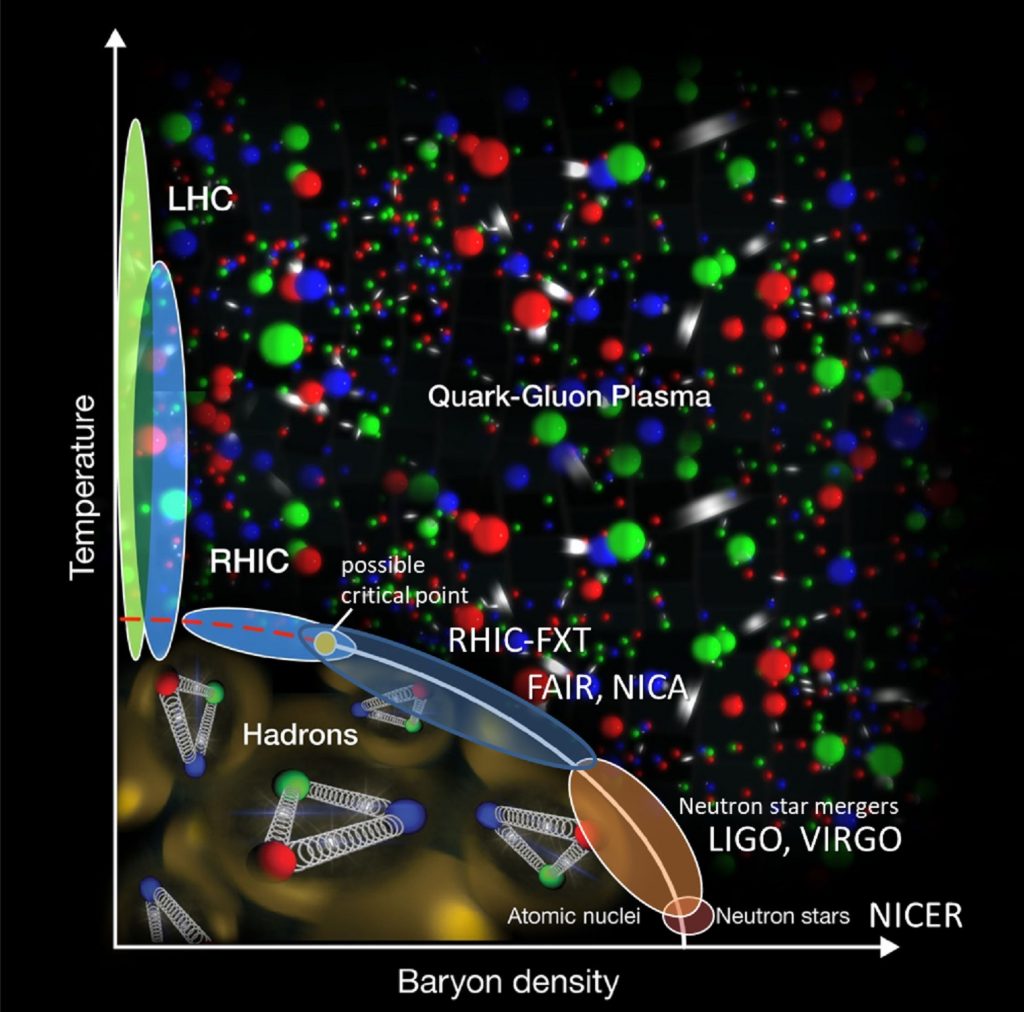
Phase diagram of nuclear matter and the facilities that can
measure the phase transition.
Enhancing Strangeness
Compared with up and down quarks, strange quarks are both far heavier, and far less stable – with any known form of matter containing the particles decaying just brief moments after their formation. Dr Bellwied and his colleagues have now discovered that during Quark Gluon Plasma re-confinement, comparatively high numbers of these quarks are created: a phenomenon known as ‘strangeness enhancement’.
‘My group established a significant enhancement in the production of strange particle, which contain strange quarks in addition to the common up and down quarks,’ Dr Bellwied illustrates. ‘These particles appear following high-speed heavy ion collisions, and can be generally considered a signature for plasma formation.’ Initially, the researchers achieved these results using collisions between heavy ions alone. Yet through subsequent research, they discovered that similar mechanisms could appear following collisions between two protons, provided the collision energy is high enough.
In parallel with these experiments, Dr Bellwied has worked with his colleague Dr Claudia Ratti, also at the University of Houston, to develop a theoretical framework for strangeness enhancement. Their theories explained how the characteristics of the re-confinement process can vary with the Quark Gluon Plasma’s temperature – revealing that strange particles will result from hotter plasmas than those that generate protons and neutrons. In subsequent research, these ideas would be key to determining how matter behaves in some of the most extreme conditions found in the known universe today.
Glimpsing the Interiors of Neutron Stars
When stars between 10 and 25 times the mass of our Sun reach the end of their lives, they will explode in dramatic supernovae, leaving behind dark, rapidly spinning cores named ‘neutron stars’. Although they measure just tens of kilometres across, neutron stars have similar masses to the Sun, making them unimaginably dense. On its surface, a neutron star appears to be composed mostly of vast numbers of highly compacted neutrons, resembling a giant atomic nucleus. However, what lies beneath the surface has largely remained a mystery so far.
Currently, the most promising techniques for studying these interiors lie in the detection of gravitational waves. These ripples in spacetime are created as colliding neutron stars spiral into each other, and eventually merge together. ‘We have now shown that high-speed collisions at lower energies generate the same energy density as those measured in neutron star mergers, as detected by gravitational waves,’ Dr Bellwied explains. ‘The makeup of the neutron star interior should generate a unique signature in the emitted wave.’
Some theorists now suggest that the extreme masses of neutron stars could be due to an abundance of stable strange quarks hidden deep in their interiors. In future research, the signatures generated by Dr Bellwied’s team could lead to experimental proof of this theory. If correct, it would suggest that hot Quark Gluon Plasmas are generated when massive stars collapse. Furthermore, this line of research may even lead to new insights into the formation of yet more enigmatic black holes.
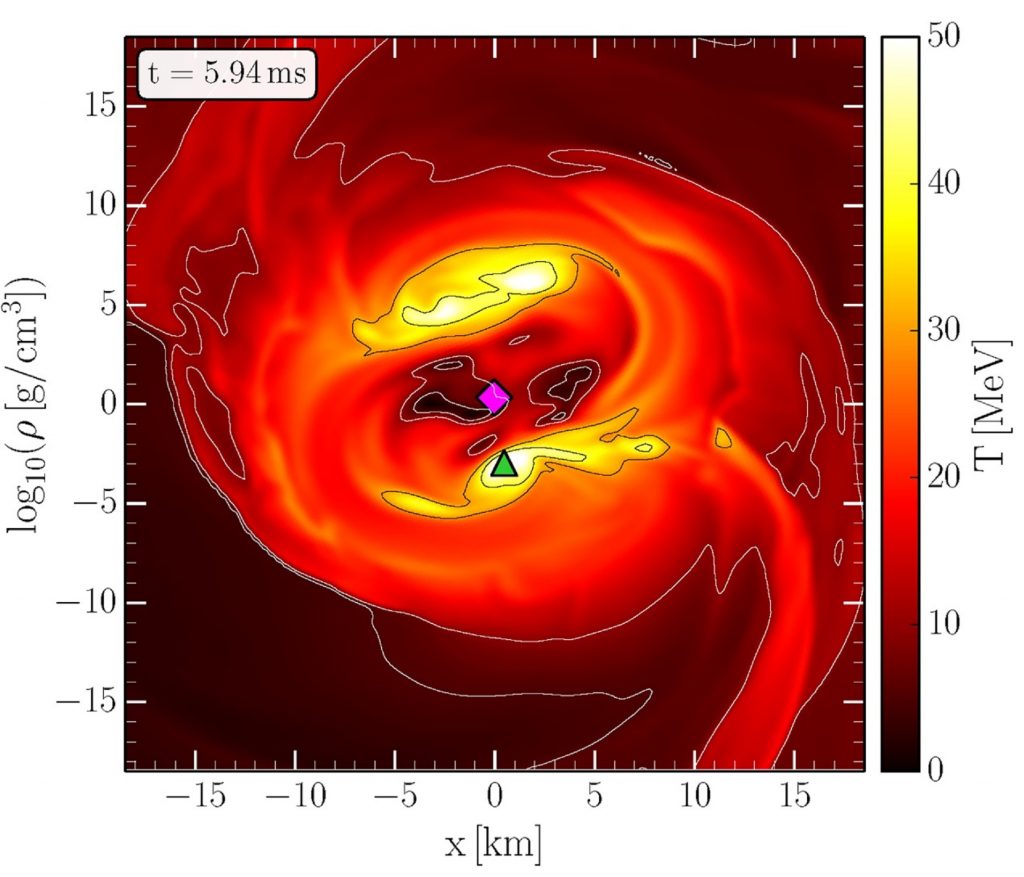
Energy profile of a neutron star merger. Particles 2, 1, 44.
The Influence of Entanglement
Through his latest research, Dr Bellwied has begun to explore how the dynamics of Quark Gluon Plasmas are influenced by the phenomenon of quantum entanglement. The effect describes systems of one or more particles whose fates are intrinsically bound to each other – so that if their states are measured at the same time, the outcomes of all observations will directly correspond to each other, no matter how far apart in space the particles are separated.
In a system of quantum particles as deeply complex as a Quark Gluon Plasma, quantum entanglement creates an intricate web of interactions between constituent quarks and gluons – strongly influencing their dynamics. Until now, however, this influence has not been widely considered. ‘I have started to look at the effects of quantum entanglement on the initial state of the plasma formation, and how it might affect the production and emission of the final state particles,’ says Dr Bellwied. ‘This is the first time that we have applied quantum mechanical principles to the evolution of deconfined matter.’
Dr Bellwied hopes that these results will lead to new insights both into the behaviours of unconfined quarks and gluons, and the nature of the matter produced following re-confinement. In turn, the findings could benefit his team’s other fields of research: including strangeness enhancement, neutron star composition, and even the fundamental properties of quarks and gluons.
Expanding the Scope for Exploration
The research of Dr Bellwied and his colleagues has already yielded important advances in our understanding of Quark Gluon Plasma and its re-confinement. However, there is still an expansive scope for future research. In the coming years, the Relativistic Heavy Ion Collider and Large Hadron Collider will be joined by two other cutting-edge particle accelerators: the Facility for Antiproton and Ion Research (FAIR) in Germany; and the Nuclotron-based Ion Collider Facility (NICA) in Russia. Even further into the future, Brookhaven National Laboratory is planning to expand its collider facilities with an Electron-Ion Collider (EIC), and Dr Bellwied’s team has recently been asked to join a collaboration to prepare the next generation detector and physics program for the EIC.
Through the more extensive collision measurements enabled by these additions, physicists may soon gain an even more advanced understanding of the dynamics that shaped our universe in its very first moments.
Reference
https://doi.org/10.33548/SCIENTIA713
Meet the researcher
Dr Rene Bellwied
Physics Department
University of Houston
Houston, TX
USA
Dr Rene Bellwied completed his PhD in Chemistry and Physics at Johannes Gutenberg University in Germany in 1989. After a Feodor Lynen Fellowship at Stony Brook University in New York, he moved to Detroit, where he worked as a Professor for 18 years at Wayne State University. In 2010, he became a Professor at the Physics Department at the University of Houston. His main research interests lie in experimental nuclear physics, and he has contributed to research into relativistic heavy ion physics at both CERN and Brookhaven National Laboratory for more than 30 years. Dr Bellwied has received much recognition for his ground-breaking research, including a Distinguished MD Anderson Endowed Chair and the 2020 University of Houston Research Excellence Award.
CONTACT
E: bellwied@uh.edu
W: https://uh.edu/nsm/physics/people/profiles/rene-bellwied/
KEY COLLABORATORS
STAR Collaboration at BNL
ALICE Collaboration at CERN
Professor Ratti, University of Houston
Professors Borsanyi and Fodor, Wuppertal University
FUNDING
US Department of Energy, Office of Science
FURTHER READING
R Bellwied, Quantum entanglement in the initial and final state of relativistic heavy ion collisions, In Journal of Physics: Conference Series (Vol. 1070, No. 1, p. 012001), 2018, IOP Publishing.
ALICE collaboration, Enhanced production of multi-strange hadrons in high-multiplicity proton-proton collisions, Nature Physics, 2017, 13, 535.
R Bellwied, S Borsanyi, Z Fodor, SD Katz, C Ratti, Is there a flavor hierarchy in the deconfinement transition of QCD?, Physical review letters, 2013, 111, 202302.
STAR collaboration, Experimental and theoretical challenges in the search for the quark–gluon plasma: The STAR Collaboration’s critical assessment of the evidence from RHIC collisions, Nuclear Physics A, 2005, 757, 102.
Want to republish our articles?
We encourage all formats of sharing and republishing of our articles. Whether you want to host on your website, publication or blog, we welcome this. Find out more
Creative Commons Licence
(CC BY 4.0)
This work is licensed under a Creative Commons Attribution 4.0 International License.
What does this mean?
Share: You can copy and redistribute the material in any medium or format
Adapt: You can change, and build upon the material for any purpose, even commercially.
Credit: You must give appropriate credit, provide a link to the license, and indicate if changes were made.
More articles you may like
Grandmothers: Innovation Through Tradition
Grandmother Project – Change through Culture (GMP) is an organisation dedicated to documenting the role of grandmothers and demonstrating the effectiveness of grandmother-inclusive strategies in improving the health and well-being of women, children, and adolescents. GMP’s groundbreaking work challenges conventional wisdom to transform community-based interventions in Africa and beyond, harnessing a powerful but often overlooked resource: the wisdom and influence of grandmothers.
Dr Robert Larkin | Cultivating Change to Improve Soil Health and Increase Potato Yield
Environmental quality and food production are facing the pressing challenges of climate change and global population growth. Dr Robert Larkin from the United States Department of Agriculture-Agricultural Research Service (USDA-ARS) and a team of plant scientists developed and tested a range of crop management systems to help overcome these compounding challenges. Their work is improving soil health and increasing the yield of potato crops, contributing to the future food security of nations.
Professor Giorgio Buttazzo | Artificial Intelligence and a Crossroads for Humanity
Where do we stand with artificial intelligence? Might machines take over our jobs? Can machines become conscious? Might we be harmed by robots? What is the future of humanity? Professor Giorgio Buttazzo of Scuola Superiore Sant’Anna is an expert in artificial intelligence and neural networks. In a recent publication, he provides considered insights into some of the most pressing questions surrounding artificial intelligence and humanity.
Dr Ralf Adam | New Technologies Shaping the Future of Oral Hygiene
Understanding the efficiency of various toothbrush technologies is essential for achieving optimal oral health. Dr Ralf Adam, who leads a dedicated team at Procter & Gamble in Germany, is keen to investigate the complexities of these technologies. His team have provided new insights into the best toothbrush types for plaque removal and the maintenance of gum health. By highlighting the importance of informed oral care decisions and ongoing investigations, this vital research works towards ensuring everyone can achieve a brighter, healthier smile.