Fine-tuning Polymers as Flexible Antenna Substrates for Aircraft
The pursuit to design more lightweight aircraft that can travel over greater distances never ceases. One area that has received much attention is the development of lighter and more aerodynamic antennas. Researchers at the NASA Glenn Research Center are at the forefront of this effort. The research team, which includes Jessica Cashman, Baochau Nguyen, Haiquan Guo, Marcos Pantoja, Kevin Cavicchi, and Mary Ann Meador, is designing flexible polymer materials that can be used to construct lightweight, low-drag antennas that lie along the surface of an aircraft.
Surface Conforming Antennas
Many unmanned aircraft communicate using large dish antennas. These antennas can be unnecessarily heavy and bulky, and project far above the surface of the vehicle, creating added drag, effectively adding extra weight. A certain type of antenna, known as a phased array antenna, can address these drawbacks. Phased array antennas are clusters of antennas that direct signals electronically without physically moving and can be installed conformally along the curved surface of a wing or fuselage.
The development of phased array antennas has been on-going. They are made up of an array of antenna elements separated by a low electrical loss material, known as a substrate. The antenna substrate needs to be readily installable at any given location of the vehicle where the surface is almost always curved. In addition, the substrate should possess electrical characteristics that do not impede signal transmission. Researchers at the NASA Glenn Research Center in the US, are developing materials that can be fabricated as flexible substrates suitable for building antennas.
The research team focuses on developing and evaluating polyimide aerogels, which have both the mechanical and electrical properties needed to build suitable antennas. Aerogels are unique in that they have one of the lowest dielectric constants of any structural material, meaning that they do not interfere with the antenna’s ability to transmit signals, allowing for the maximum amount of data to be transferred. These materials also have very small pores, meaning that they can be viewed by the antenna elements as a solid substrate.
Polyimides are polymers, that is, they are made up of many (poly) repeating carbon-containing units (mers) connected to form a backbone. The chemical structure of the backbone influences the stiffness of the polymer, as well as other properties. Polyimides are typically rigid backbone polymers, since the mers contain less flexible ring structures.
Preparing Polymer Substrates
The preparation of polyimide aerogels involves several stages. First, the polymer precursors (monomers) are dissolved in a solvent and immediately react to form linear chains. Then a crosslinker is added, which reacts with the chains forms a network structure. This solution is poured into moulds before the network formation is complete. As network formation goes to completion, a gel is formed where the solvent remains trapped within the polymer network. Consequently, when the solvent is removed, it leaves behind pores in the material where pockets of solvent had been. The resulting aerogel material possesses an extremely nano-porous structure.
Polymer aerogels can be viewed as a complex network of overlapping and interlocking molecular chains. The density of a polymer aerogel can be controlled by the amount of molecules in solution, but is also influenced by how much shrinkage occurs during processing. The denser the aerogel, the more it will resist physical deformation and the stiffer the material will be. However, the less dense the aerogel is, the less it will interfere with the antenna.
NASA’s polymer aerogel team can fine-tune how the chains pack together by changing the structures and ratios of the repeating groups of molecules along the polymer backbone. To develop the best possible antenna substrate, the researchers have been optimising the mechanical properties such as the flexibility, pore size, and water resistance of polyimide aerogels, by incorporating flexible links alternating with units of rigid polyimide. They aim to achieve an aerogel material with the lowest possible density, and thus dielectric constant, combined with an optimum flexibility.
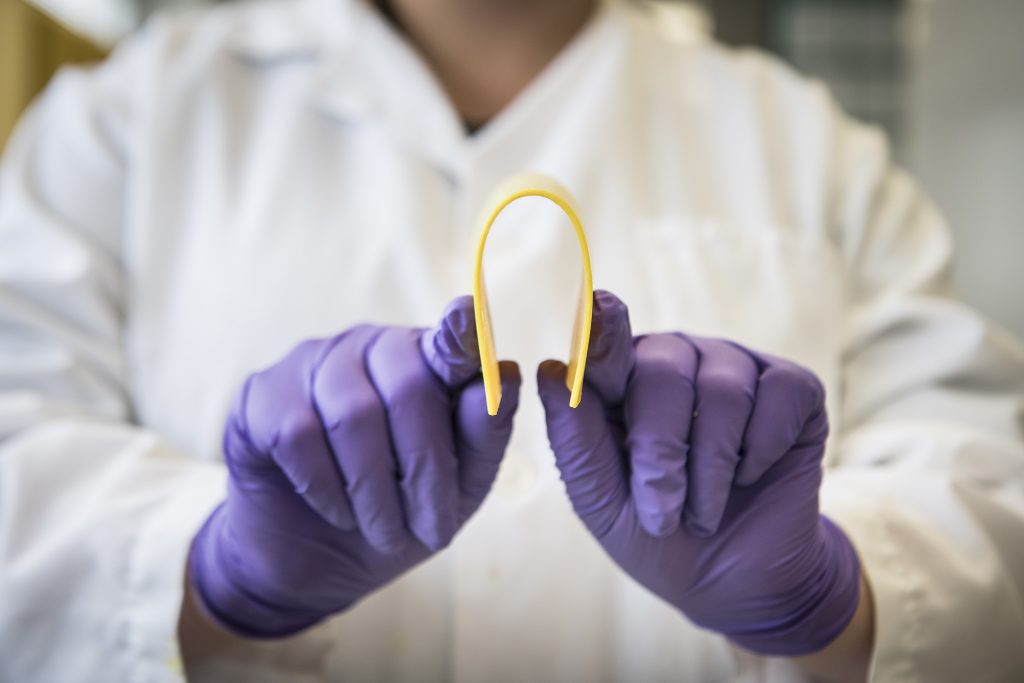
Reprinted with permission from Pantoja, M.; Boynton, N.; Cavicchi, K.; Dosa, B.; Cashman, J. L.; Meador, M. A. B. Increased Flexibility in Polyimide Aerogels Using Aliphatic Spacers in the Polymer Backbone, ACS Appl. Mater. Interfaces 2019, 11, 9. Copyright 2019 American Chemical Society.
Effects of Flexible Linking Group Length
The research team began their studies by incorporating a flexible linking group into the backbone of the polyimide polymer and investigating how the length of the linking group affects the physical properties of the polyimide aerogel. Polyimide is a rigid polymer and adding the flexible linking groups to the backbone is what makes the polymer more flexible. They also explored the proportions of reagents needed to alter the backbone, and how these ratios ultimately affected the aerogel’s physical properties.
Specifically, they investigated how the number of methylene groups used to vary the length of the flexible linking group can affect the characteristics of the material. Since methylene groups form a linear chain, they allow the chain to fold easily. An analogy to this is a beaded necklace. The rigid polyimide units are like the beads and the flexible chains of methylene groups are like the thread between the beads. The team compared different sizes of the flexible linking groups with four, six and ten methylene groups.
Their results revealed that the length of the flexible linker positively influenced some of the material properties, while also proving problematic for others. The following summarises the results gleaned from the study.
The team found that the flexibility of the aerogel increased when at least 25% of the flexible linker was added, but the length of the methylene chains did not have much of an influence on flexibility. In addition, using greater than 25% flexible linkers caused more shrinkage, leading to higher density aerogels. Interestingly, they were able to demonstrate how a two-millimetre-thick aerogel substrate could be bent so that the folds were about two inches apart.
The researchers also investigated whether their aerogel materials would absorb water, which would short the antenna elements. Fortunately, they showed that aerogels made up of longer methylene chains absorb very little water. From this result and the above mechanical properties, the team concluded that 25% of longer flexible linking group resulted in the optimum material.
In a more recent study, the researchers performed a similar analysis with polymer compositions containing 12 methylene groups. They achieved similar flexibility using this spacer, but the material’s resistance to water was even better.
Studying Bulkier Backbones
Continuing the overall theme of their previous work, the team then investigated the utility of a different chemical group when altering the backbone. In this study, they compared the effect of neopentyl groups with their work involving methylene groups. Neopentyl is flexible because it consists of a three-carbon chain between two ether groups. However, the middle carbon contains two methyl groups, which makes it more moisture resistant.
The researchers investigated how different proportions of reagents needed to incorporate neopentyl groups into the polymer chains affect the structure of the polymer, and ultimately, the mechanical properties of the aerogel. They also compared some of the key results between their methylene and neopentyl studies.
When the solvent is removed from a wet gel to form an aerogel, the structure shrinks. Minimising shrinkage of the aerogel is desired in order to maintain a low density and dielectric constant. The researchers found that aerogels shrank the most when they had added a large amount of neopentyl-containing monomer during the synthesis.
They were also able to derive other trends when higher proportions of neopentyl-containing monomer were introduced. For example, more sophisticated experimental methods that approximate the surface area of the dried aerogel revealed that the surface area of the porous aerogel decreased. This relates quite well with the shrinkage of the aerogel. As the aerogel shrinks, the pores collapse, and the available surface area decreases.
They also analysed the ability of the aerogel to absorb water and found that adding 50% of neopentyl-containing monomer resulted in minimal water uptake. If the proportion was reduced or increased from the optimal point, then the aerogel would absorb more water.
As shown in their methylene study, the researchers demonstrated how a two-millimetre-thick film of the neopentyl-based aerogel could be bent to within a couple of inches. Overall, the researchers concluded that adding 50% of neopentyl containing monomer would yield an aerogel with optimal flexibility, combined with a low dielectric constant.
The work carried out by NASA’s polymer aerogel team was intricate and challenging. Nonetheless, understanding the relationships between atomic-level attributes and large-scale mechanical properties proved invaluable in their search for the most suitable conformal antenna substrates. In fact, in all three of the studies described here, the team achieved desirable flexibility in each optimised material, making them ideal for use in the manufacture of lightweight, high-performing antennas for more efficient unmanned aircraft.
Reference
https://doi.org/10.33548/SCIENTIA668
Meet the team
Dr Haiquan Guo
Universities Space Research Association
NASA Glenn Research Center
Cleveland, OH
USA
Dr Haiquan Guo received her PhD in Chemistry at City University of New York in 2004. In 2007, she began working at Ohio Aerospace Institute in Cleveland, and currently works at Universities Space Research Association as a senior scientist. She researches the development of polymer aerogels and inorganic aerogels for aerospace and aeronautic applications.
E: haiquan.n.guo@nasa.gov
Dr Marcos Pantoja
Boeing Research and Technology
Saint Louis, MO
USA
Marcos Pantoja received his PhD in Polymer Engineering from The University of Akron. Part of his studies involved the synthesis and evaluation of flexible polyimide aerogels through a joint research effort with NASA Glenn Research Center. Marcos is now a Materials and Process Engineer at the Boeing Research and Technology organisation, where he researches different rate enabling manufacturing processes focused on polymer processing in support of Boeing commercial and defence platforms.
E: marcos.pantoja@boeing.com
Jessica Cashman
NASA Glenn Research Center
Cleveland, OH
USA
Jessica Cashman received her BS in Chemical Engineering at Rochester Institute of Technology in 2018. Following graduation, she started working for NASA Glenn Research Center in Cleveland. She initially focused her research in polymer and inorganic aerogels for aerospace and aeronautic applications and now works on power and energy storage systems for extreme environments.
E: Jessica.l.cashman@nasa.gov
Dr Baochau Nguyen
Universities Space Research Association
NASA Glenn Research Center
Cleveland, OH
USA
Dr Baochau Nguyen completed her PhD in Polymer Science at the University of Akron. She then worked at Ashland Chemical Company, Ohio Aerospace Institute and currently, at the University Space and Research Association. Her research involves synthesis, processing and characterisation of different polymers and composites, aerogels, battery membranes and ceramics.
E: Baochau.N.Nguyen@nasa.gov
Dr Mary Ann Meador
Meador Aerospace Materials Group
Johns Island, SC
USA
Mary Ann Meador received her BS in chemistry in 1979 from Duquesne University and her PhD in organic chemistry in 1984 from Michigan State University. In her 35-year career at NASA Glenn Research Center, Dr Meador has published over 200 articles and 33 patents in the areas of high temperature polymers, polymer electrolytes, and polymer aerogels. She is currently CEO of Meador Aerospace Materials Group LLC, and an adjunct Professor of Polymer Engineering at the University of Akron.
E: mmeador@uakron.edu
Dr Kevin Cavicchi
The University of Akron
Akron, OH
USA
Kevin Cavicchi is a Professor of Polymer Engineering at the School of Polymer Science and Polymer Engineering at the University of Akron. His research group is interested in the structure-property-processing relationships of nanostructured soft-matter including small molecule organogels, ionomers, block copolymers, and their blends using commercial and custom synthesised polymers prepared by free-radical, reversible addition fragmentation chain transfer (RAFT), and anionic polymerisation.
E: kac58@uakron.edu
FUNDING
NASA – Transformative Aeronautics Concepts Program under the Convergent Aeronautic Solutions subproject: Conformal Lightweight Antenna Systems for Aeronautical Communication Technologies (CLAS – ACT)
FURTHER READING
H Guo, MAB Meador, JL Cashman, D Tresp, B Dosa, DA Scheiman, LS McCorkle, Flexible Polyimide Aerogels with Dodecane Links in the Backbone Structure, American Chemical Society Applied Polymer Materials, 2020, 12, 33288–33296.
JL Cashman, BN Nguyen, B Dosa and MAB Meador, Flexible Polyimide Aerogels Derived from the Use of a Neopentyl Spacer in the Backbone, American Chemical Society Applied Polymer Materials, 2020, 2, 2179–2189.
M Pantoja, N Boynton, KA Cavicchi, B Dosa, JL Cashman, MAB Meador, Increased Flexibility in Polyimide Aerogels Using Aliphatic Spacers in the Polymer Backbone, American Chemical Society Applied Materials and Interfaces, 2019, 11, 9425–9437.
Want to republish our articles?
We encourage all formats of sharing and republishing of our articles. Whether you want to host on your website, publication or blog, we welcome this. Find out more
Creative Commons Licence
(CC BY 4.0)
This work is licensed under a Creative Commons Attribution 4.0 International License.
What does this mean?
Share: You can copy and redistribute the material in any medium or format
Adapt: You can change, and build upon the material for any purpose, even commercially.
Credit: You must give appropriate credit, provide a link to the license, and indicate if changes were made.
More articles you may like
Dr Timothy Beers | Mapping the Galaxy’s Stellar Populations Using Large Photometric and Astrometric Surveys
Astronomers often use spectroscopic (electromagnetic radiation) data and astrometric (motion and positional) data to develop working models describing our Galaxy. Dr Timothy Beers from the University of Notre Dame and his collaborators in Korea and China combined large photometric (visible light) surveys and astrometric data to create multidimensional maps of a large part of the Galaxy. By highlighting significant inhomogeneities in stellar-chemical compositions, motions, and spatial distributions, Dr Beers and his colleagues provide valuable insights into how we can advance our understanding of the formation and evolution of our Galaxy.
Dr Anushia Inthiran | Distance Learning: Impacts for Offshore Students Amid COVID-19
The COVID-19 pandemic significantly disrupted global education and necessitated a shift to online learning. Due to ongoing border closures, even after the pandemic eased, offshore students were prevented from attending their university in person long after their local peers, impacting their learning ability and future perspectives. Dr Anushia Inthiran from the University of Canterbury conducted a survey among a group of offshore students to understand the consequences of distance learning on their education.
Dr Sébastien Weber | PyMoDAQ: Navigating the Future of Data Acquisition
In an era where data is paramount, Dr Sébastien Weber and his team at CNRS, the French National Centre for Scientific Research, are changing the landscape for scientists and engineers with PyMoDAQ, an open-source data acquisition software. Their revolutionary tool stands out for its accessibility, versatility, and the thriving community it fosters.
Professor Gary Yohe | Navigating Climate Change: The Impactful Contributions of Gary Yohe
Professor Gary Yohe is a distinguished environmental economist whose work has been pivotal in shaping our understanding of climate change impacts, adaptation strategies, and policy frameworks. His interdisciplinary approach combines economics with environmental science, offering nuanced insights into global warming and its multifaceted impacts on natural and human systems. Professor Yohe equips us with the knowledge and strategies needed to navigate the complex and pressing challenges posed by climate change.