Professor Lijun Wang – Developing Transportable and High-performance Atomic Clocks
By measuring the frequencies emitted as atoms transition between energy levels, atomic clocks are among the most advanced devices available for keeping time. In his research, Professor Lijun Wang at Tsinghua University, Beijing, explores how the stringent requirements for these devices can be met using easily transportable apparatus. By combining the latest technological advances in ion trapping, laser cooling, and magnetic shielding, his team has now achieved a design that far exceeds the performance of existing transportable clocks – potentially leading to new capabilities for both high-speed scientific measurements, precision time-keeping, and applications in satellite-based navigation.
Atomic Clocks
To measure the passage of time, clocks rely on uniformly oscillating systems named ‘frequency standards’. Whether they use the swinging pendulum in a grandfather clock or the pulsating quartz in an electrical watch, all timekeeping devices can only register that time has passed after their frequency standards have completed a full cycle of oscillation. Ultimately, this means that a clock’s accuracy is inherently limited by the uniformity in time between its frequency standard’s oscillations.
In recent decades, cutting-edge devices named ‘atomic clocks’ have allowed many researchers to keep time far more accurately than ever before. They operate by measuring the highly consistent frequencies of electromagnetic radiation, emitted when atoms in excited states transition back into lower-energy states. The technology typically relies on bulky, importable apparatus – but Professor Lijun Wang’s team at Tsinghua University shows how high-performing atomic clocks could soon become far more compact and easier to transport. Through a series of experiments, his team proposes alternatives to existing setups that could make them far easier to apply in real-world scenarios.
Hyperfine Structures
Because of the laws of quantum mechanics, all atoms only possess certain discrete values of energy, named ‘energy levels’ – and cannot take on any energies lying between these points. To transition between energy levels, atoms emit and absorb photons of light with energies, and therefore frequencies, identical to the difference in energy between them. There are several different types of energy levels an atom can possess: with the largest gaps between values leading to characteristically different orbital patterns of an atom’s outer electron. On the other end, energy levels with the smallest energy differences between them can be described by an effect named ‘hyperfine structure’.
Within an atom, the interaction between the atomic nuclear spin, and that of the orbiting electrons, leads to a ’hyperfine interaction’, which depends on the spins’ relative orientation. Since each individual moment has a tiny yet discrete energy value, an atom’s larger-scale energy levels can essentially be ‘split’ into several, very slightly different energy levels. Crucially, atoms can transition between these levels by emitting or absorbing low-energy photons, whose frequencies lie in the microwave region of the electromagnetic spectrum.
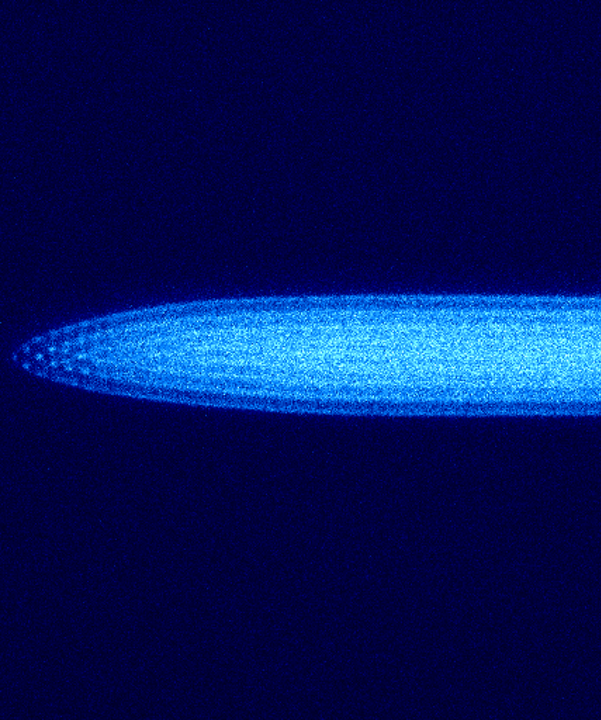
Cadmium ion crystal
Developing Frequency Standards
Since the energy levels associated with an atom’s hyperfine structure are so close together and involve only very weak interaction, the frequencies of the microwave radiation emitted during transitions are extremely precise. As a result, these photons make for a highly effective frequency standard in atomic clocks – which would need to keep time for several months before being out by just a single nanosecond. To operate, microwave atomic clocks use lasers to excite ultra-cold atoms to higher-energy hyperfine states, then measure the frequencies of the microwaves they emit as they transition back to lower energy levels.
These systems can be highly effective in principle – but the technology still faces significant challenges. Because the microwave signals produced during hyperfine transitions are so extremely subtle, they must be measured to extreme degrees of accuracy, with virtually no room for error. In recent years, researchers have achieved this level of precision by trapping ultra-cold ionised atoms using applied external radiation fields. So far, this has allowed physicists to precisely identify the transition frequencies of ions including mercury, barium, beryllium, and ytterbium. In a series of studies published between 2015 and 2021, Professor Wang’s team studied a more promising, cadmium ion with high precision for the first time.
Exploiting a Simple Structure
A key advantage of using trapped ions in atomic clocks stems from their long lifetimes. This allows them to interact with the applied microwave radiation field over extended periods of time – making the ions suitable for tightly controlled measurements of hyperfine transition frequencies.
Professor Wang and his colleagues used this technique on ions of cadmium isotope 113. They chose this element and isotope because when a single electron is removed from its outer shell, its lowest-energy ‘ground’ state has an advantageous, relatively simple hyperfine structure – which isn’t split between too many different energy levels and is less susceptible to the residual external magnetic field.
This feature makes cadmium ions ideal for the technique of ‘laser cooling’: which exploits momentum changes during photon emission and absorption to cool atoms to close to absolute zero, minimising any fluctuations in their positions. At the same time, the laser can be used to excite ions to higher energy levels and read out the frequencies they emit as they transition back to lower energy levels. Although the transition frequencies of several other ions are also known to have extremely high degrees of precision, the simplicity of cadmium’s ground-state hyperfine structure and particularly the need for only one laser presents a unique potential for realising miniaturised, highly practical atomic clocks.
Establishing Precision
Despite its clear advantages as a frequency standard, the hyperfine structure of the cadmium ion had not yet been explored in detail. For their experiments, Professor Wang’s team made their measurements using a device named a ‘Paul trap’, which rigidly confines charged particles using oscillating electric fields. Combining the traps with laser cooling, the researchers investigated two different ionised isotopes of cadmium: 113Cd+ and 111Cd+. Through a 2012 study, they used these combined techniques to assess the hyperfine transition frequencies of both ions, producing values several orders of magnitude more precise than had ever been achieved previously.
In a series of subsequent studies, the team improved on this precision even further. By 2015, they had reached a fractional precision of 10-14 – meaning their measured frequency was correct to within just several 100 trillionths of its actual value. Building on these achievements, Professor Wang and colleagues next aimed to demonstrate the use of these hyperfine cadmium transitions as a practical frequency standard. However, they still faced several challenges before this goal could be realised.
Sympathetic Cooling
Altogether, the process of obtaining a frequency standard from an atomic clock is composed of three steps: preparing ions in confined, ultra-cold states; exciting them to higher energy levels with a laser; and finally, the detection of their transition frequency.
Ultimately, the need for the first two steps in this cycle limits the amount of time spent actually measuring the transition frequency – resulting in a lack of information that limits the stability of the frequency standard in the short term. Furthermore, with the improvement of external field shielding technology, the uncertainty caused by the thermal movement of the ions has become the main limitation preventing further improvements in accuracy. To overcome these issues, Professor Wang and his colleagues turned to the technique of ‘sympathetic cooling’.
Here, ions that are cooled directly by laser cooling are themselves used to cool other ions indirectly, as they exchange momentum through mutual Coulomb interactions. In a 2019 study, the researchers showed that when parameters were optimised, cadmium ions could be cooled to temperatures as low as 0.2 degrees above absolute zero, through sympathetic cooling of magnesium ions confined within a Paul trap. Without the need to prepare the cadmium ions directly, they essentially cut out the first step of the frequency measurement cycle – suppressing any dead time and improving the stability of the resulting frequency standard in turn.
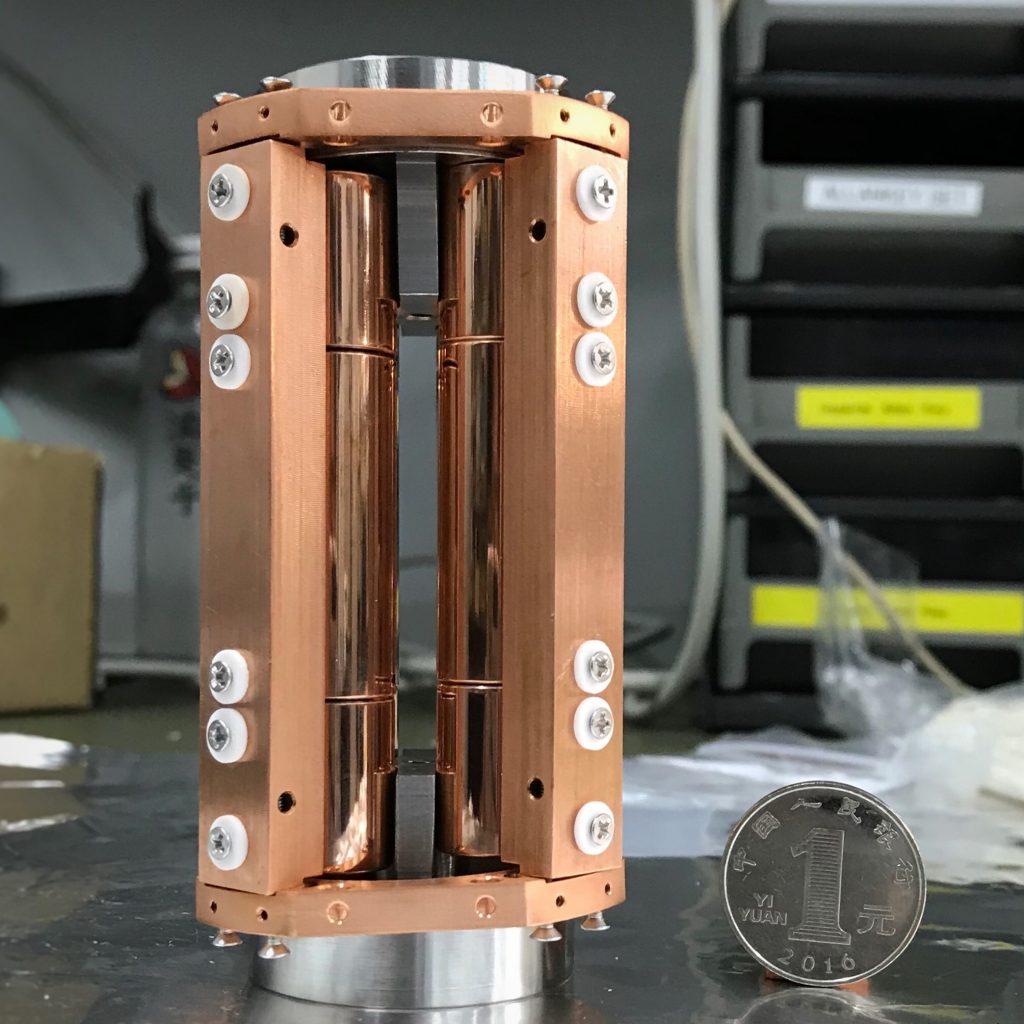
Paul trap
A Transportable Microwave Clock
With this technological adjustment in place, Professor Wang’s team was finally ready to develop a high-performance, transportable microwave atomic clock, suitable for real-world applications. In their latest 2021 study, the researchers confined a system of 100,000 113Cd+ ions within a Paul trap, and sympathetically cooled them to temperatures just 0.09 degrees above absolute zero using, calcium ions. In addition, they addressed problems stemming from two inconvenient shifting effects – which arise from unpredictable interactions between ions and magnetic fields and can slightly distort the frequencies measured by a laser.
By surrounding their setup with five layers of ‘magnetic shield’ material, the team significantly suppressed the strength of the magnetic field passing through the system, improving the precision of its measurements even further. Measuring just 1.4 cubic metres in volume, the apparatus was small enough to fit on a tabletop. At the same time, it could produce a microwave frequency with fractional uncertainties on the order of 10-15, while being easily transportable by ground-based vehicles. Through the success of their demonstration, Professor Wang and his colleagues now envisage numerous uses for their clock, which go beyond the capabilities of existing, far bulkier setups with similar performances.
Expanding Applications in Timekeeping
Ever since their conception, the unprecedented timekeeping ability of atomic clocks has opened up numerous new possibilities for scientific experiments, and even technologies we use in our everyday lives. With the ability to easily move the apparatus around, these applications could be extended further still. For researchers, they could improve the ability to measure physical processes which play out on extremely small timescales: including interactions between quantum particles within complex materials; collisions within particle accelerators; and energetic astronomical events.
Elsewhere, the technology could prove invaluable to satellite-based navigation. These systems operate by measuring the exact time taken for light to travel between a target object, and a number of different satellites at known positions in Earth’s orbit – allowing them to triangulate the object’s position to pinpoint precision. Highly precise, portable atomic clocks will further afford higher precision in satellite position measurement and tracking. Improvements in the atomic clock equipment could improve our navigational ability in scenarios ranging from the daily commute to deep space exploration.
Reference
https://doi.org/10.33548/SCIENTIA734
Meet the researcher
Professor Lijun Wang
Department of Physics and Department of Precision Instruments
Tsinghua University
Beijing
China
Professor Lijun Wang received his PhD in Physics at the University of Rochester, New York, in 1992. In 2004, he was invited to set up the Max Planck Institute for the Science of Light jointly at the University of Erlangen-Nuremberg in Germany, where he also worked as a chair professor for experimental physics. Professor Wang has served as a professor in both the Department of Physics and the Department of Precision Instruments at Tsinghua University since 2008. His research interests lie in precision measurement and instrumentation. Professor Wang’s current areas of research include ion trapping for higher-precision atomic clocks, measuring the strength of Earth’s gravity, and ultra-high precision time and frequency synchronisation and dissemination. He is Fellow of the Optical Society of America (OSA), the American Physical Society (APS) and the National Institute of Metrology of China.
CONTACT
E: lwan@mail.tsinghua.edu.cn
KEY COLLABORATORS
Dr Jianwei Zhang, Tsinghua University
Dr Jize Han, Tsinghua University
FUNDING
National Key R&D Program of China (No. 2016YFA0302101)
Major State Basic Research Development Program of China (973 Program, No. 2010CB922901)
FURTHER READING
JZ Han, HR Qin, NC Xin, YM Yu, VA Dzuba, JW Zhang, LJ Wang, Toward a high-performance transportable microwave frequency standard based on sympathetically cooled 113Cd+ ions, Applied Physics Letters, 2021, 118, 101103.
YN Zuo, JZ Han, JW Zhang, LJ Wang, Direct temperature determination of a sympathetically cooled large 113Cd+ ion crystal for a microwave clock, Applied Physics Letters, 2019, 115, 061103.
K Miao, JW Zhang, XL Sun, SG Wang, AM Zhang, K Liang, LJ Wang, High accuracy measurement of the ground-state hyperfine splitting in a 113Cd+ microwave clock, Optics Letters, 2015, 40, 4249.
JW Zhang, SG Wang, K Miao, ZB Wang, LJ Wang, Toward a transportable microwave frequency standard based on laser-cooled 113Cd+ ions, Applied Physics B, 2014, 114, 183.
SG Wang, JW Zhang, K Miao, ZB Wang, LJ Wang, High-accuracy measurement of the 113Cd+ ground-state hyperfine splitting at the milli-Hertz level, Optics Express, 2013, 21, 12434.
JW Zhang, ZB Wang, SG Wang, K Miao, B Wang, LJ Wang, High-resolution laser microwave double-resonance spectroscopy of hyperfine splitting of trapped 113Cd+ and 111Cd+ ions, Physical Review A, 2012, 86, 022523.
Want to republish our articles?
We encourage all formats of sharing and republishing of our articles. Whether you want to host on your website, publication or blog, we welcome this. Find out more
Creative Commons Licence
(CC BY 4.0)
This work is licensed under a Creative Commons Attribution 4.0 International License.
What does this mean?
Share: You can copy and redistribute the material in any medium or format
Adapt: You can change, and build upon the material for any purpose, even commercially.
Credit: You must give appropriate credit, provide a link to the license, and indicate if changes were made.
More articles you may like
Grandmothers: Innovation Through Tradition
Grandmother Project – Change through Culture (GMP) is an organisation dedicated to documenting the role of grandmothers and demonstrating the effectiveness of grandmother-inclusive strategies in improving the health and well-being of women, children, and adolescents. GMP’s groundbreaking work challenges conventional wisdom to transform community-based interventions in Africa and beyond, harnessing a powerful but often overlooked resource: the wisdom and influence of grandmothers.
Dr Robert Larkin | Cultivating Change to Improve Soil Health and Increase Potato Yield
Environmental quality and food production are facing the pressing challenges of climate change and global population growth. Dr Robert Larkin from the United States Department of Agriculture-Agricultural Research Service (USDA-ARS) and a team of plant scientists developed and tested a range of crop management systems to help overcome these compounding challenges. Their work is improving soil health and increasing the yield of potato crops, contributing to the future food security of nations.
Professor Giorgio Buttazzo | Artificial Intelligence and a Crossroads for Humanity
Where do we stand with artificial intelligence? Might machines take over our jobs? Can machines become conscious? Might we be harmed by robots? What is the future of humanity? Professor Giorgio Buttazzo of Scuola Superiore Sant’Anna is an expert in artificial intelligence and neural networks. In a recent publication, he provides considered insights into some of the most pressing questions surrounding artificial intelligence and humanity.
Dr Ralf Adam | New Technologies Shaping the Future of Oral Hygiene
Understanding the efficiency of various toothbrush technologies is essential for achieving optimal oral health. Dr Ralf Adam, who leads a dedicated team at Procter & Gamble in Germany, is keen to investigate the complexities of these technologies. His team have provided new insights into the best toothbrush types for plaque removal and the maintenance of gum health. By highlighting the importance of informed oral care decisions and ongoing investigations, this vital research works towards ensuring everyone can achieve a brighter, healthier smile.