Dr Chao Sun – The Complex Relationships Behind Tumour Progression in Cancer
The processes and metabolic pathways in our cells are complex and extensive, but essential for normal life functions, including cell respiration and energy production via the mitochondria. However, sometimes these processes go wrong resulting in disease, including cancer. Dr Chao Sun from the Institute of Modern Physics, Chinese Academy of Sciences, dedicates his research to unravelling the mechanisms behind tumour progression in relation to mitochondrial dysfunction.
Mitochondria: The Powerhouse of the Cell That Can Go Wrong
Within every cell, there are tiny structures that work together to keep it alive and functioning. These structures are known as organelles and the many different types each have their own purpose to fulfil. One such organelle is the mitochondrion, which is commonly referred to as the powerhouse of the cell. The reason for this is because it produces the energy needed to power the processes and biochemical reactions in the cell, in the form of adenosine triphosphate (ATP) molecules. Starting with a glucose molecule, ATP is synthesised through a series of chemical reactions called the Krebs cycle, or the citric acid cycle. The glucose needed for this energy creation comes from the food we eat and this cycle is an important part of aerobic respiration – creating energy with glucose and oxygen.
In addition to creating energy, mitochondria are involved in other important cell processes. They absorb and store calcium for when it is needed and can even generate heat when body temperature is too low. Although they take part in processes necessary for life, they also take part in cell death, known as apoptosis. They help to decide when a cell dies by releasing a chemical that activates the caspase enzyme which breaks down the cell from the inside.
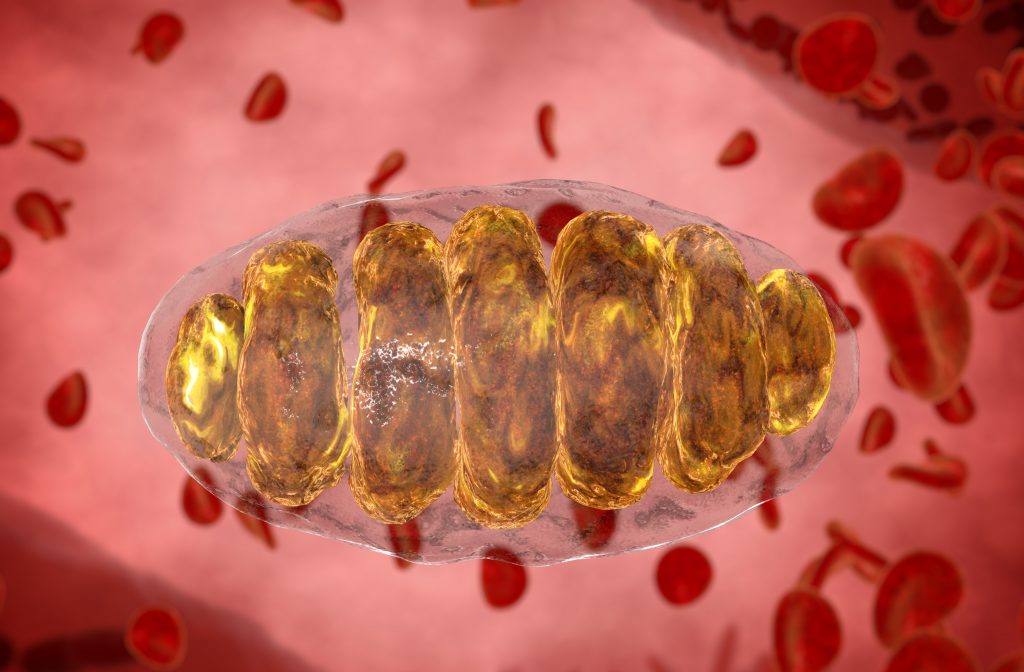
Mitochondrion
The unique structure of the mitochondria, and the smaller structures within, help them to carry out their jobs effectively. Like only two other organelles, the mitochondria have two membranes surrounding them and the folds of the inner membrane, called cristae, increase its surface area to allow more space for ATP creation. Sized between 0.75 and 3 micrometres, mitochondria can be found in varying numbers depending on the cell type. Mature red blood cells have none at all, whereas liver cells have more than 2,000. Usually, cells that require more energy have a higher number of mitochondria.
In human body cells, only two organelles hold DNA – most are in the nucleus but a small amount is stored in the mitochondria. However, it is more susceptible to damage and consequent disease here due to molecules called reactive oxygen species, or free radicals, that are a by-product of ATP synthesis. Mitochondrial diseases are also caused by spontaneous or inherited mutations in nuclear DNA that result in faulty proteins within the mitochondria. If this means that it cannot function and create sufficient energy for the cell, it may cause a variety of different symptoms, depending on which cell is affected.
Muscle weakness, vision and hearing issues, neurological and gastrointestinal problems can all arise thanks to mitochondrial dysfunction. Additionally, there is evidence that Parkinson’s disease, chronic fatigue syndrome and even cancer may involve malfunctioning mitochondria.
Hypoxia and Disease
Cancer in relation to mitochondrial dysfunction is the focus of Dr Chao Sun’s research at the Institute of Modern Physics, Chinese Academy of Sciences in Lanzhou, China. He also links these processes to hypoxia and molecules called hypoxia-inducible factors (HIFs). These are a type of transcription factor, which are proteins that control the process of DNA being decoded to eventually create new proteins. HIFs respond to a decrease in oxygen within the cell, known as hypoxia.
This environment can be a result of a variety of issues like anaemia and heart and lung diseases preventing oxygen from getting around the body properly. However, hypoxia can also be caused by structural and functional problems of the cell and organelles. When the mitochondria are malfunctioning, respiration decreases because less oxygen is available and other important pathways to maintain a healthy cell are disrupted.
When HIFs are activated by hypoxia, they participate in reactions related to metabolism, proliferation and angiogenesis. This means they take part in biochemical reactions for cell life, cell multiplication and the formation of new blood vessels. These are all important factors that allow cancer, the abnormal and uncontrolled growth of cells, to develop. ‘We systematically discuss the crosstalk between HIFs and mitochondrial dysfunctions in cancer development’, says Dr Sun, in consideration of the interesting results arising from his studies.
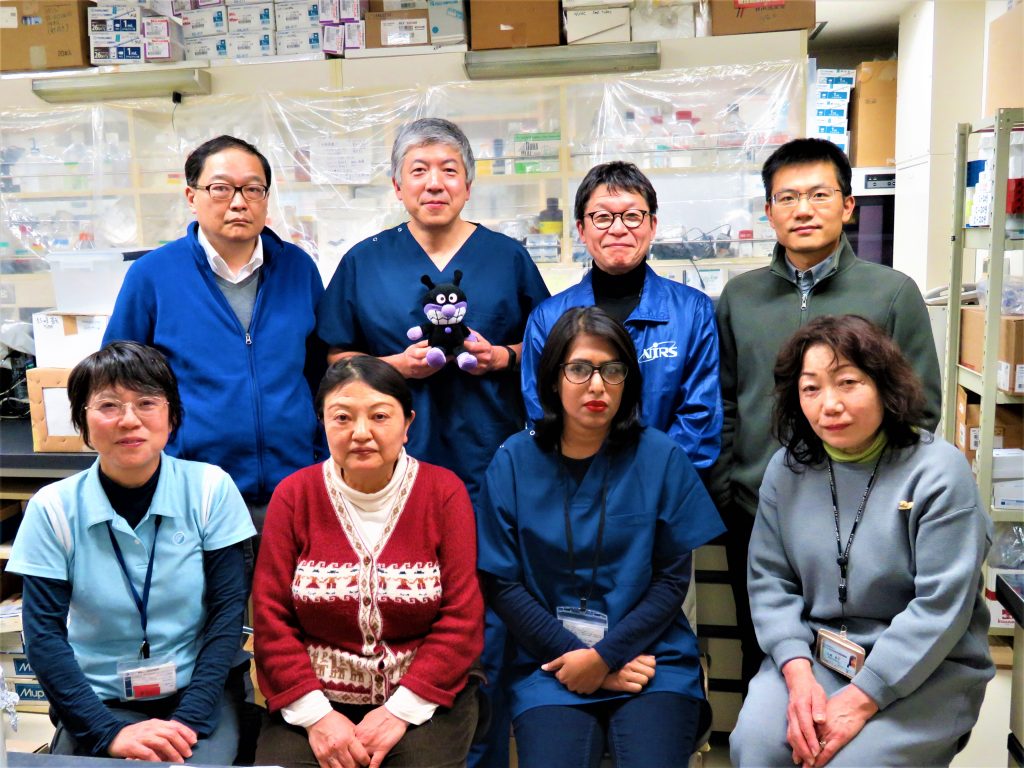
Dr Sun and his team at the National Institutes for Quantum and Radiological Science and Technology, Japan
Proton Displacement in Adenosine Triphosphate Synthesis
In order for ATP synthesis to be possible, protons (positively-charged hydrogen atoms) are pumped into the space between the membranes of the mitochondria, called the intermembrane space. This creates a strong electrochemical gradient (mitochondrial membrane potential, MMP) across the inner membrane. A constant MMP means that protons pass back through via the enzyme ATP synthase which consequently makes energy. Normal physiological functioning and sufficient energy supply for the cell requires a maintained MMP.
An early study by Dr Sun investigated how an anti-oxidant drug called MitoQ interacts with this process. The purpose of MitoQ is to reduce the number of reactive oxygen species in the mitochondria and it is being tested as a therapy for a number of diseases from Parkinson’s to heart disease. A section of the MitoQ molecule is positively charged and it remains in the intermembrane space, making the mitochondria act as though this space is full of protons.
Dr Sun found that to keep the MMP balanced, the organelle reacts by producing fewer protons and decreasing the proton pumping rate. However, this disrupts the normal ATP production and supply, so he dubbed the phenomenon pseudo-MMP (PMMP). With the mitochondria no longer able to cope with cell energy and respiratory demand, the cell begins to degrade itself in a process called autophagy.
These were exciting results from Dr Sun, because they were the first to talk about PMMP, the mechanisms by which it occurs and how autophagy takes place when protons are displaced by other positive charges. This gives insight into why the drug may be effective for killing cancer cells.
Mitochondrial Transplantation as a Cancer Therapy
A subsequent study by Dr Sun and his colleagues looked into how mitochondrial dysfunction and consequent disturbances to energy metabolism are associated with cancer. These links can be seen in cancer cells with mitochondrial dysfunction which causes an increase in glucose breakdown for energy (glycolysis), decreased cell death and resistance to radiotherapy.
The team transplanted mitochondria from healthy cells into brain cancer cells and starved them of glucose. They found that this slowed down the rate of glycolysis and decreased the number of protons available in the cells. The transplant also enhanced gene and protein expression related to the Krebs cycle, meaning more of the proteins necessary for the pathway were created, so respiration increased. It also reactivated the process in the mitochondria that encourages cell death, whilst inhibiting unwanted multiplication of the cancer cell.
In tests with mice, Dr Sun discovered that when healthy mitochondria were injected into tumours, they successfully entered the cancer cells through endocytosis. As a result, the healthy mitochondria inhibited the growth of the tumour and he believes they could make tumours more sensitive to the destructive effects of radiotherapy. These are exciting findings because they are the first to demonstrate mitochondrial transplantation as a potential therapy for tumours, including those that are resistant to radiotherapy.
Dr Sun proposes that mitochondria could be taken from healthy cells of a cancer patient, processed outside of the body and then reintroduced to their tumour. If successful, this could be a promising and fascinating intervention as it would be highly specific to and effective for the patient, without the risk of immune rejection.
A Complex Relationship
Shifting his focus to HIFs in relation to cancer, Dr Sun and his team conducted a systematic review of the evidence for a relationship between HIFs and mitochondrial dysfunction in cancer development. They described how cancer cells are reliant on changes to normal metabolism that preserve energy, support cell growth and produce molecules that encourage tumours to thrive. Understanding how these adaptations come about is essential to discover weaknesses in pathways that could be exploited with treatments.
As previously mentioned, there is extensive evidence that HIFs have a role in regulating pathways in cancer and Dr Sun paired this understanding with the Warburg effect. This is a hallmark of tumours whereby metabolic adaptations, such as high glucose uptake through glycolysis, help cancer cells to survive in hypoxia. A product of this high glucose uptake, lactate, induces the production of HIFs and the hypoxic conditions activate the molecules. Both HIFs and mitochondrial dysfunction cause energy metabolism to be altered and are, therefore, very likely connected. Dr Sun explains that ‘activation of HIFs can lead to mitochondrial dysfunction by affecting multiple mitochondrial functions including mitochondrial oxidative capacity, biogenesis, apoptosis, fission, and autophagy’.
As a whole, Dr Sun’s review demonstrates that tumours developing and growing is in part due to an ‘extensive and cooperative network’ of mitochondrial dysfunctions and HIFs. Looking to the future, he believes that further elucidating these complex relationships through additional research will aid the progression of diagnostics and therapeutics for cancer patients worldwide.
SHARE
DOWNLOAD E-BOOK
REFERENCE
https://doi.org/10.33548/SCIENTIA756
MEET THE RESEARCHER

Dr Sun and his team at the Chinese Academy of Science, China
Dr Chao Sun
Institute of Modern Physics
Chinese Academy of Sciences
Lanzhou
China
Dr Chao Sun received his undergraduate degree in Pharmacology and his Master of Medicine from Shihezi University in Xinjiang, China. He then achieved a PhD in Biophysics from the Institute of Modern Physics, Chinese Academy of Sciences in Lanzhou, China. Currently, this is where Dr Sun is a senior professor and also where he carries out his research. His work involves investigating mitochondrial dysfunction and how it can be involved in cancer development. In addition to his teaching and research duties, Dr Sun is also a guest associate editor for the scientific journal, Frontiers in Public Health. At present, Dr Sun is also a collaborative researcher of National Institutes for Quantum and Radiological Science and Technology, Japan, and serves as a visiting professor at Yantai University, China.
Contact
E: sunchao@impcas.ac.cn
W: http://people.ucas.edu.cn/~sunchao13?language=en
Key Collaborators
Qiang Li, Institute of Modern Physics, Chinese Academy of Sciences, China
Hong Zhang, Institute of Modern Physics, Chinese Academy of Sciences, China
Bing Wang, National Institutes for Quantum and Radiological Science and Technology, Japan
Katusbe Takanori, National Institutes for Quantum and Radiological Science and Technology, Japan
Xiongxiong Liu, Institute of Modern Physics, Chinese Academy of Sciences, China
Xingting Bao, Institute of Modern Physics, Chinese Academy of Sciences, China
Zhenhua Wang, Yantai University, China
Funding
The Youth Innovation Promotion Association of the Chinese Academy of Sciences (2020414)
The National Natural Science Foundation of China (11775280)
The National Natural Science Foundation of China (11505245)
The Chinese Academy of Sciences ‘Light of West China’ Program (Y866020XB0)
The Natural Science Foundation of Gansu Province (18JR3RA391)
Further Reading
X Bao, J Zhang, G Huang, et al., The crosstalk between HIFs and mitochondrial dysfunctions in cancer development, Cell Death & Disease, 2021, 12(2), 1–13.
C Sun, X Liu, B Wang, et al., Endocytosis-mediated mitochondrial transplantation: Transferring normal human astrocytic mitochondria into glioma cells rescues aerobic respiration and enhances radiosensitivity, Theranostics, 2019, 9(12), 3595.
C Sun, X Liu, C Di, et al., MitoQ regulates autophagy by inducing a pseudomitochondrial membrane potential, Autophagy, 2017, 13(4), 730–738.
REPUBLISH OUR ARTICLES
We encourage all formats of sharing and republishing of our articles. Whether you want to host on your website, publication or blog, we welcome this. Find out more
Creative Commons Licence (CC BY 4.0)
This work is licensed under a Creative Commons Attribution 4.0 International License.
What does this mean?
Share: You can copy and redistribute the material in any medium or format
Adapt: You can change, and build upon the material for any purpose, even commercially.
Credit: You must give appropriate credit, provide a link to the license, and indicate if changes were made.
SUBSCRIBE NOW
Follow Us
MORE ARTICLES YOU MAY LIKE
Dr Ralf Adam | New Technologies Shaping the Future of Oral Hygiene
Understanding the efficiency of various toothbrush technologies is essential for achieving optimal oral health. Dr Ralf Adam, who leads a dedicated team at Procter & Gamble in Germany, is keen to investigate the complexities of these technologies. His team have provided new insights into the best toothbrush types for plaque removal and the maintenance of gum health. By highlighting the importance of informed oral care decisions and ongoing investigations, this vital research works towards ensuring everyone can achieve a brighter, healthier smile.
Professor Alan Templeton | When Fire Sparks Ecological Opportunity and Habitat Restoration
How far would you be willing to go to save an endangered species? Would you consider burning part of a forest as a solution? As unconventional as it may sound, conservationists sometimes resort to such measures to restore lost habitats. One remarkable example is the efforts to save eastern collared lizards – and indeed the entire biological community in which they live – in the Ozarks, spearheaded by American geneticist and statistician Professor Alan Templeton of Washington University in St Louis, USA.
Dr Toby Phesse | Revealing the Mysteries of Wnt Signalling: Novel Approaches to Beating Cancer
Cancer remains a leading cause of mortality worldwide, and the need for new, more effective treatments remains an urgent challenge. Dr Toby Phesse from Cardiff University in the UK focuses on the role of the Wnt receptor found on the surface of cells and its involvement with cell communication and cancer growth, bringing fresh hopes for new therapeutic options.
Dr Vijay Reddy | The Virus World Database: An Invaluable Resource for Public Health and Healthcare
Severe viral disease presents an ongoing challenge to the health of humankind. While unparalleled developments in science and technology are improving our understanding of such viruses, this information needs to be readily accessible to researchers to ensure continued progress in public health and healthcare. Dr Vijay Reddy and his colleagues at the Hormel Institute (University of Minnesota) developed the Virus World database, an invaluable resource that details the genome, structure, and host of practically every discovered virus to date.