Dr Michael E. Green – Alisher M. Kariev | Understanding How Charged Particles Cross Living Cell Membranes
Living organisms function through a complex interplay of cellular mechanisms. The movement of charged atoms across cell membranes is key to diverse processes like cell growth and electrical signalling in the brain, and channels in these membranes open and close to allow charged atoms to pass through. Dr Michael Green and Alisher M. Kariev at the City College of the City University of New York challenge current models of channel opening and closing to provide a more complete understanding of this crucial and widespread biochemical process.
Building Blocks of Life
Proteins, one of the main biological molecules, are giant molecules that may contain electrical charges and may rearrange their structure. Voltage-gated ion channels are proteins with pores that open and close with a gate in response to changes in the voltage in a cell membrane, allowing the passage of certain charged atoms or ions.
Ion channels are present in the entire range of known lifeforms and perform the key function of moving ions across a cell membrane. The membrane separates the water-like inside and outside of the cell with an oil-like barrier through which ions cannot pass, meaning that a channel is needed to provide a tightly controlled path for the ions. Voltage-gated potassium channels allow the rapid and selective flow of potassium ions through cell membranes, and are vital to cell growth and differentiation, hormone release, maintaining cardiac activity, and the electrical signalling of brain and nervous-system cells such as neurons.
A great deal is already known about these channels: they are made up of four voltage-sensing domains, each with four segments that span the membrane, and these have electrical charges. Two segments from each of these voltage-sensing domains form a joint central conduction pore through which ions can travel. The voltage sensors rearrange with changing membrane voltage, altering the conduction channel either structure or charge distribution, and leading to either opening or closing of the ion conduction pathway gate. When the membrane is polarised, with positive charges on one side and negative charges on the other, the gate remains closed, and as ions cannot otherwise pass through membranes, this gating mechanism stops ion traffic.
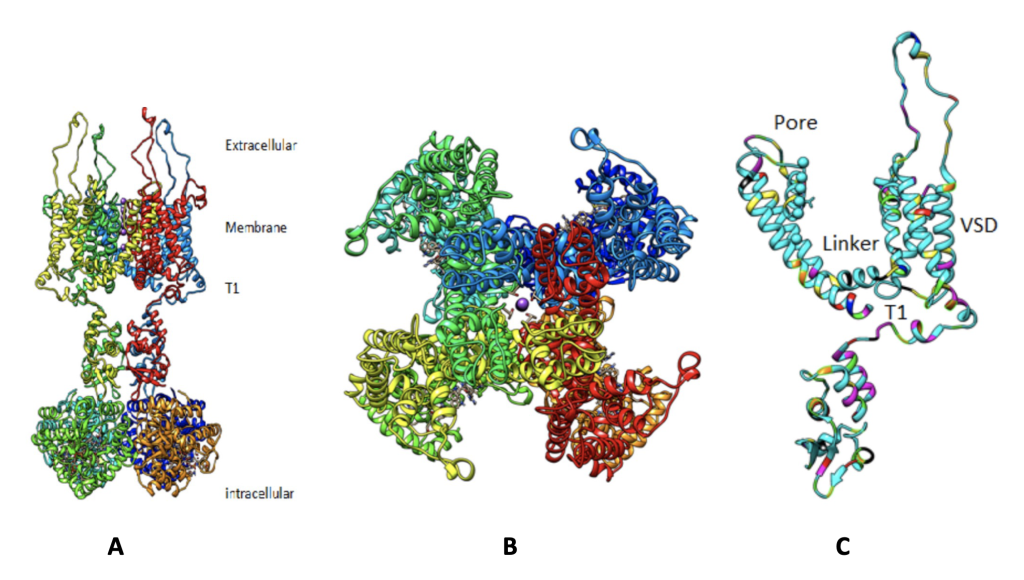
Figure 1: A, B: Two views of the channel protein: A: looking sideways, inside the cell at the bottom, the potassium path upward from there to the outside. The gate is at the bottom of the membrane section, just above the level labeled T1. B: View from outside the cell, with the central pore being the potassium path, and the colored sections at the outside the voltage sensing domains. C: The linker connecting the voltage sensing domain and the gate, expanded view. The voltage-gated potassium channel. Reproduced with permission of Michael Green from https://doi.org/10.3390/membranes12070718 under a Creative Commons Attribution license.
Credit: Michael Green.
Challenging Conventional Models
Gates in the channels inside the cell membranes open and close to let ions pass – but how and why? Although charges clearly move when a channel opens, researchers are unsure of the exact mechanism involved.
As voltage gating in ion channels is such a key process in living systems, various models have been proposed to explain it. The accepted conventional model describes particular membrane segments that move to open the channel because of a change in the distribution of electrical charge. According to this idea, these same segments then move in the opposite direction when the membrane returns to its initial charge distribution.
Dr Michael Green and Alisher Kariev at the City College of the City University of New York suggest that caution may be required when interpreting evidence that has been taken to support the conventional approach, which attributes the movement of charges occurring when gates open to extensive rearrangements of membrane sections. ‘No complete model of such a rearrangement has been found, and no conclusive experiment exists,’ says Dr Green.
From Structural Shifts to Moving Charges
The researchers developed an alternative model to explain ion channel gating, in which mobile charges within the membrane are explained in terms of the transfer of protons – stable subatomic particles with a positive electrical charge.
They focused their investigations on the path taken by protons, making the assumption that these are the moving charges in the channel. ‘This is the controversy – most people believe the charges are not protons,’ adds Dr Green. ‘But the standard model has never been completed, with no version being consistent with all the data.’
The researchers aimed to provide a clear and robust description of this key phenomenon, describing new proton paths in voltage-gated potassium channels. ‘It is part of a model of ion channel gating that is interesting in that it differs from the most common models,’ adds Dr Green. ‘This is part of a project intended to determine whether protons could be the source of the gating current in ion channels.’
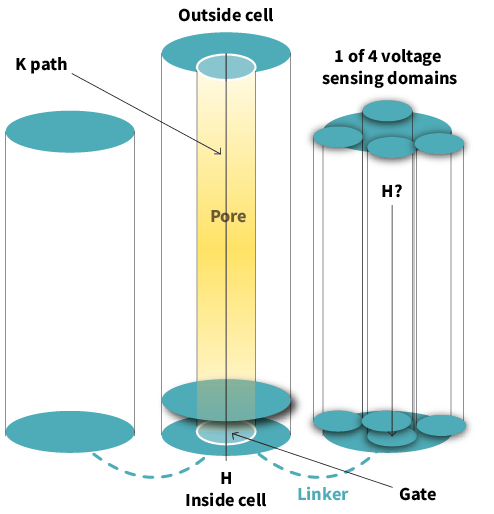
A schematic diagram of an ion channel that responds to voltage by opening to transmit potassium ions (K) from the inside to the outside of the cell.
Credit: Michael Green
Atomic-level calculations
The need to shed light on the underlying processes involved in channel gating prompted the team to use detailed computational techniques to calculate the properties of an ion channel. They used high-precision atomic-level computer modelling tools to obtain the structure, charge, and energy of a simulated channel system.
Dr Green and Alisher Kariev’s hunt for the optimal properties of their model channel began with a computer model of an open-gated channel made of over 1,300 atoms, including several water molecules, which was built according to a structure that had been experimentally determined using X-rays.
The researchers added two protons to the model using a technique that describes the different flexible states that are accessible to proteins that are in an equilibrium state.
The rapid movement of hydrogen ions along a chain of hydrogen-bonded water molecules – known as a proton wire – is, in the Green-Kariev model, an important mechanism in channels, and the team found this effect in the portion of the channel running between the voltage sensor and gate.
Discovering New Proton Paths
Their precise atomic-level calculations provided the researchers with a set of optimised atomic structures for a voltage-gated potassium channel, complete with details on energy and electronic charges. By observing the right kind of proton-transmitting residues at each end of the computed path, the team was able to show that the channel gate, which opened and closed to let ions pass, was connected to voltage sensors by proton paths. They uncovered two clearly defined proton paths between the voltage sensors and gate, and discovered that a crossover path between these also existed.
The gating concept proposed by Dr Green and Alisher Kariev opens up the potential to develop more detailed models to describe ion channels. While the researchers state that further calculations are needed to completely understand gating mechanisms, they have successfully shown that protons can traverse a key section of a voltage-gated potassium channel, shedding light on a process occurring in almost every living organism on the planet.
SHARE
DOWNLOAD E-BOOK
REFERENCE
https://doi.org/10.33548/SCIENTIA1011
MEET THE RESEARCHER
Dr Michael E. Green
Department of Chemistry and Biochemistry
The City College of the City University of New York
New York, NY
USA
Dr Michael Green graduated from Cornell University with a BS in chemistry, followed by an MSc and PhD in physical chemistry at Yale University. After undertaking postdoctoral research at the California Institute of Technology, Dr Green spent two years teaching at Middle East Technical University as a Peace Corps volunteer, after which he joined the faculty of City College of the City University of New York, initially as an assistant professor, later promoted to associate professor and then professor. His main focus is on quantum-level calculations related to chemistry and biochemistry. Over the course of his career, Dr Green has been a visiting scientist at a wide range of international institutes, including Hacettepe University in Ankara and the Lanzhou Institute of Chemical Physics. Dr Green is now Professor Emeritus at the City College of the City University of New York.
CONTACT
W: www.ccny.cuny.edu/profiles/michael-green
Alisher M. Kariev
Department of Chemistry and Biochemistry
The City College of the City University of New York
New York, NY
USA
Alisher Kariev obtained his BS in cybernetics at the Tashkent Institute of National Economy, and then moved to the Faculty of Engineering Physics at Tashkent Polytechnical Institute to complete his MSc. He undertook further postgraduate studies in biophysics at the Academy of Sciences, Kyiv, and specialised in quantum chemical studies at the Ukraine Institute of Physical Chemistry and the Ukraine Institute of Theoretical Physics. After working at the Academy of Science, Moscow, with Professor Ovchinnikov, and the Cybernetics Institute of the Science Academy of Uzbekistan, Alisher Kariev took up his current position as a research associate at The City College of the City University of New York. Here, he focuses on the state and role of water in biological ultrastructures such as potassium channels, and quantum calculations on potassium channel selectivity and gating.
CONTACT
KEY COLLABORATORS
Roman Shirikov, Rutgers University, USA
FURTHER READING
AM Kariev, ME Green, Protons in Gating the Kv1.2 Channel: A Calculated Set of Protonation States in Response to Polarization/Depolarization of the Channel, with the Complete Proposed Proton Path from Voltage Sensing Domain to Gate, Membranes, 2022, 12(7), 718. DOI: https://doi.org/10.3390/membranes12070718
REPUBLISH OUR ARTICLES
We encourage all formats of sharing and republishing of our articles. Whether you want to host on your website, publication or blog, we welcome this. Find out more
Creative Commons Licence (CC BY 4.0)
This work is licensed under a Creative Commons Attribution 4.0 International License.
What does this mean?
Share: You can copy and redistribute the material in any medium or format
Adapt: You can change, and build upon the material for any purpose, even commercially.
Credit: You must give appropriate credit, provide a link to the license, and indicate if changes were made.
SUBSCRIBE NOW
Follow Us
MORE ARTICLES YOU MAY LIKE
Professor Alan Templeton | When Fire Sparks Ecological Opportunity and Habitat Restoration
How far would you be willing to go to save an endangered species? Would you consider burning part of a forest as a solution? As unconventional as it may sound, conservationists sometimes resort to such measures to restore lost habitats. One remarkable example is the efforts to save eastern collared lizards – and indeed the entire biological community in which they live – in the Ozarks, spearheaded by American geneticist and statistician Professor Alan Templeton of Washington University in St Louis, USA.
Dr Michael Cherney – Professor Daniel Fisher | Unlocking Woolly Mammoth Mysteries: Tusks as Hormone Time Capsules
The impressive tusks found on proboscideans (the order of mammals that includes elephants, woolly mammoths, and mastodons) are like time capsules, preserving detailed records of their bearers’ lives in the form of growth layers and chemical traces. Frozen in time for thousands of years, these layers can unlock secrets about the lives of long-extinct relatives of modern elephants. Dr Michael Cherney and Professor Daniel Fisher from the University of Michigan used innovative techniques to extract and analyse steroid hormones preserved in woolly mammoth tusks. This ground-breaking work opens new avenues for exploring the biology and behaviour of extinct species.
Dr Sebastian Fraune | Microbiota: Fast-tracking Adaptation to Rapidly Changing Environments
As climate change continues at an unprecedented pace, the processes of natural selection and genetic mutation can no longer fully explain how some organisms adapt to their rapidly changing environments. Dr Sebastian Fraune from Heinrich-Heine University and an international team of researchers are the first to demonstrate a causal relationship between changes in the microbiome and changes in thermal tolerance. They propose that microbiota-mediated transgenerational acclimatisation can account for how animals adapt to their environments in much shorter periods of time than classical theory would predict.
Professor Danny Reinberg – Dr Gary LeRoy | Confronting a Rare and Deadly Cancer in Children
A diagnosis of cancer is never welcome but is particularly devastating when a child is found to have diffuse intrinsic pontine gliomas – a rare but particularly aggressive and treatment-resistant malignancy in the brain. In this article, we focus on the contributions of Professor Danny Reinberg and Dr Gary LeRoy at the University of Miami-Miller School of Medicine to understanding what makes these tumours so dangerous and, importantly, to developing much-needed approaches to improve the outcomes for these children.