Professor Alison Lister | Poking Holes in the Standard Model of Particle Physics
Our understanding of physics changed dramatically in the 20th century, with the advent of the Standard Model of Particle Physics, which builds on quantum mechanics and Einstein’s theory of relativity – two of the most successful theories in the history of science. However, we know that our theories are incomplete, but finding out what’s beyond the Standard Model is difficult because it’s such a successful theory. Professor Alison Lister and her colleagues at the University of British Columbia and around the world are poking holes in the Standard Model, towards finding a new theory that gives a more complete description of the universe.
Challenging Science’s Most Successful Theory
Modern physics is in a strange position. In the 20th century, our understanding of physics changed dramatically. Einstein’s theories of special and general relativity provided us with deep and beautiful explanations of gravity, space, time, and the large-scale structure of the universe. Meanwhile, the emergence of quantum mechanics reshaped our understanding of the smallest structures in the universe.
The combination of these two revolutions in our understanding led to the development of Quantum Field Theory. When combined with ground-breaking experimental results, Quantum Field Theory led to the establishment of the Standard Model of Particle Physics. The Standard Model is a theory centred around the universe being made up of a small number of fundamental particles, forces and rules governing their interactions – which is the foundation of our modern understanding of physics.
The Standard Model has made testable predictions that have been verified with unprecedented accuracy. However, it is also fundamentally incompatible with general relativity, and neither are complete theories on their own.
Further, the standard model cannot explain why there is matter in the universe. Each particle of matter has a complementary anti-particle, which is identical in seemingly every way to its corresponding particle but with an opposite electric charge. When a matter particle collides with its antiparticle, the two are annihilated into pure energy. This seeming symmetry between matter and antimatter clashes with the universe we see, which is filled with stars, gas, and planets, all made of matter. The Standard Model suggests matter and antimatter should have been produced in equal amounts in the early universe, but this would lead to a universe without matter or antimatter.
Professor Alison Lister at the University of British Columbia is a world-leading experimental physicist, and an expert on the Standard Model. The goal of her research is to break it, towards developing a more complete picture of the universe.
Beyond the Standard Model
As Professor Lister argues, our best way to get past the Standard Model and find a deeper theory of physics is to ‘poke holes’ in it: find situations where the theory makes inaccurate predictions. This gives us clues about what’s wrong about the Standard Model, and also lets us constrain the theories that might replace it. These are called ‘Beyond the Standard Model’, or BSM, theories, and there are many of them, such as theories with extra space-time dimensions. Figuring out the most promising replacement is hard, precisely because the current Standard Model is so successful, and it’s difficult to devise experiments to distinguish which theory is most accurate.
Professor Lister is tackling this problem on two fronts: precision measurement and searching for particles beyond the Standard Model.
Crucial to both of these goals is the Large Hadron Collider (LHC). The LHC is a particle accelerator, located in a 27-kilometre ring-shaped tunnel that lies between France and Switzerland. This enormous contraption allows physicists to accelerate protons to near light-speed and collide them. This process is so energetic that it lets physicists probe the limits of our theories, exploring conditions that simulate extreme high-energy interactions or the first few nanoseconds after the Big Bang. At four different points around the tunnel, the beams are brought into collision in the middle of giant detectors, packed full of incredibly sensitive devices, giving physicists a window into what exactly happens after such high-energy particles collide.
Experiments at the LHC have confirmed many Standard Model predictions, most notably discovering the Higgs Boson. The Higgs Boson is a particle that was predicted by physicists developing the Standard Model in the 1970s, but wasn’t experimentally detected until 2012 at the LHC.
However, many researchers have actually been hoping to be surprised by the LHC experiments, for the reasons described above: it would give us tantalising insights into the theories beyond the Standard Model. So far, the results have been accurately predicted by the Standard Model, but Professor Lister hopes to change this.
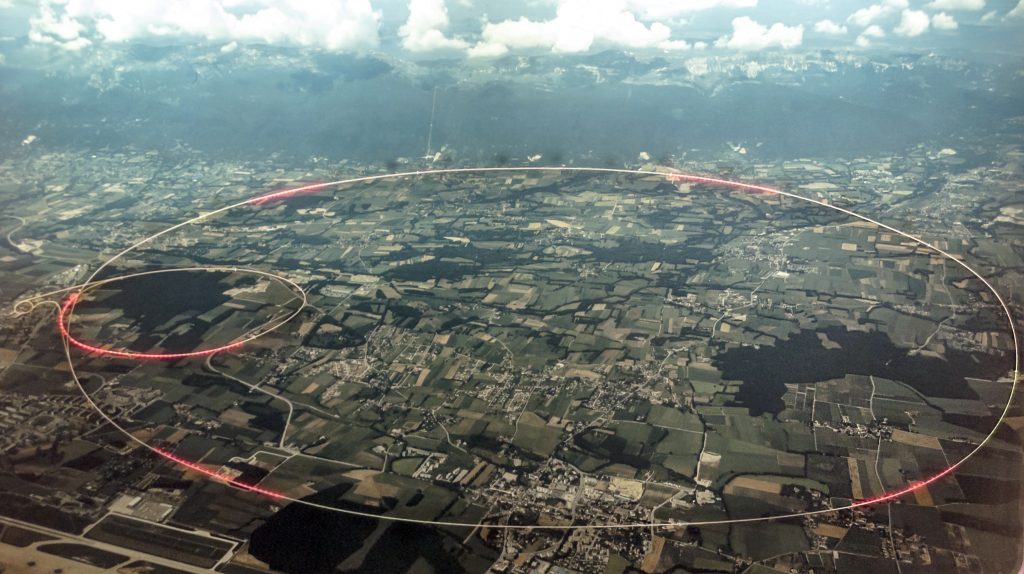
Aerial photo of the LHC
Precision Measurements on Top
By measuring experimental data, physicists can identify deviations between theory and reality. However, these deviations can sometimes be miniscule. Therefore, Professor Lister and her colleagues focus on extremely precise measurements, so that they can find these tiny deviations.
The team’s primary object of study for these precision measurements are particles called ‘top quarks’. Quarks are a type of fundamental particle that, along with leptons such as electrons, are the basis of all atomic matter in the universe. Quarks come in six varieties, or ‘flavours’, evocatively named ‘up’, ‘down’, ‘charm’, ‘ strange’, ‘top’, and ‘bottom’.
Up and down quarks are the most common, as they constitute the protons and neutrons within atoms. The other four flavours are more exotic, and Professor Lister studies the heaviest of these: top quarks. While up and down quarks weigh far less than a hydrogen atom, each top quark has the mass of an atom of gold.
Because of their large mass, top quarks are only created in extreme environments such as high-energy collisions. These do occur naturally, for example when high energy cosmic rays strike particles in the Earth’s atmosphere, but they cannot be analysed well there. Instead, physicists use the LHC to generate top quarks to measure their properties and see what happens when they decay.
Top quarks have an incredibly short lifespan, decaying on average just half a trillionth of a trillionth of a second after they’re created. You might think this makes it hard to study top quarks, but it’s actually a blessing in disguise, as it means they are the only quark that can be observed directly. Their short lifespan means that they don’t have time to combine with other quarks, like the other five quarks very quickly do. This means top quarks are the only quark that can be studied in isolation.
Further, mass is fundamentally linked to the Higgs field and thus the Higgs Boson. The Higgs field is crucial to our understanding of how and why particles have the masses they do. Thus, the large mass of top quarks renders them directly detectable and allows physicists to indirectly study the properties of the Higgs Boson, providing more opportunities to test the Standard Model and constrain the theories beyond it.
Professor Lister pioneered this type of precision measurement experiment at the LHC using ATLAS. Her goal is to improve the sensitivity and precision of the data, by improving our accuracy of correctly identifying top quarks, and correcting for impacts of the detector on the data. She has been using and developing machine-learning tools for over a decade to improve our sensitivity to BSM physics.
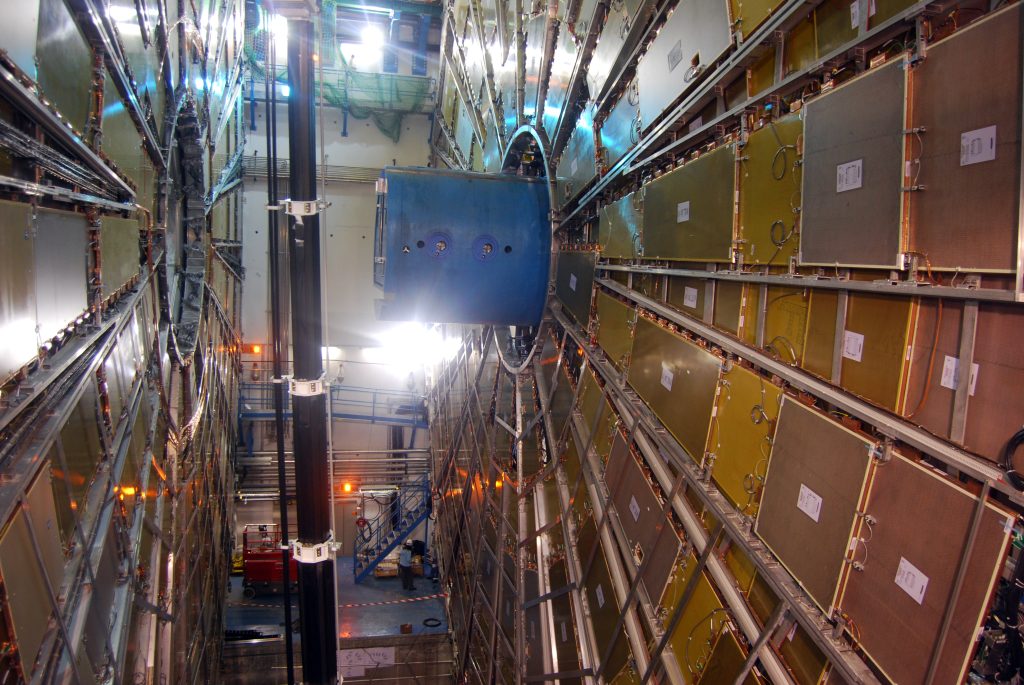
The ATLAS detector in the LHC
Searching for Long-Lived Particles
The second focus of Professor Lister’s work is searching for so-called long-lived particles.
As we discussed earlier, we know the Standard Model isn’t a complete theory. One area we know it falls short is explaining ‘dark matter’.
When astrophysicists began studying distant galaxies, they discovered a strange phenomenon: they appeared to spin faster than their apparent mass should permit. Stars at the edge of a galaxy seemed to orbit so fast that they should have been thrown out of the galaxy, and yet their orbits are stable. This suggests that galaxies contain much more mass than we can see, anchoring these stars in orbit.
Astronomers posed many explanations and refined their measurements and theories, but still failed to find a conventional source for this invisible mass. Most physicists now agree that some kind of exotic matter must contribute this mass. This is labelled dark matter, since it seemingly doesn’t interact with light.
However, the Standard Model doesn’t propose any particle that fits this description. This means that understanding and explaining dark matter will be crucial to developing theories beyond the Standard Model.
Professor Lister and her colleagues have approached this by trying to synthesise potential ‘dark matter candidates’ using the LHC. If they can produce and detect a particle that matches the properties expected for dark matter, this would be the first ever direct detection of something from beyond the Standard Model, kickstarting a whole new era of particle physics research.
Many theories beyond the Standard Model predict particles that might explain dark matter, but they do so in wildly different ways. Some predict a single particle, while others predict a whole ‘dark sector’ of currently unknown particles. Ruling these theories in or out could dramatically change our understanding of the universe, and re-prioritise global physics research.
Physicists around the world are waiting with baited breath for researchers to find flaws in the Standard Model, and no one knows whether the next big leap in our understanding of physics will come from the discovery of some new dark matter particle in the LHC, or from some tiny deviation between theory and measurement, such as in the properties of the top quark. Professor Lister and her team work on both fronts, and their research may contribute to the largest shift in our understanding of physics in a century.
SHARE
DOWNLOAD E-BOOK
REFERENCE
https://doi.org/10.33548/SCIENTIA897
MEET THE RESEARCHER
Alison Lister
Associate Professor
The University of British Columbia
Vancouver
Canada
Professor Alison Lister is a world-leading experimental physicist with over two decades of experience. In 1998, she undertook her degree in Physics at the University of Oxford in the UK, before joining ETH Zurich in Switzerland for her PhD on the measurement of b-quark jet shapes at the Collider Detector at Fermilab, obtaining her doctorate in 2006. She then continued her research at the University of California at Davis, USA, and the Université de Genève in Switzerland. In 2012, she joined the University of British Columbia in Vancouver, where she now works as an Associate Professor and Canada Research Chair in particle physics, conducting ground-breaking research, supervising a host of PhD students, and lecturing on particle physics, relativity, quantum mechanics, experimental methods and more.
CONTACT
E: alister@phas.ubc.ca
W: https://phas.ubc.ca/users/alison-lister
REPUBLISH OUR ARTICLES
We encourage all formats of sharing and republishing of our articles. Whether you want to host on your website, publication or blog, we welcome this. Find out more
Creative Commons Licence (CC BY 4.0)
This work is licensed under a Creative Commons Attribution 4.0 International License.
What does this mean?
Share: You can copy and redistribute the material in any medium or format
Adapt: You can change, and build upon the material for any purpose, even commercially.
Credit: You must give appropriate credit, provide a link to the license, and indicate if changes were made.
SUBSCRIBE NOW
Follow Us
MORE ARTICLES YOU MAY LIKE
Dr Olalla Castro-Alvaredo | Measuring Entanglement: Symmetry-Resolved Entropy
Dr Olalla Castro-Alvaredo of the City University of London (UK) and her collaborators are advancing our understanding of an important phenomenon of quantum mechanical systems known as entanglement and, especially, its mathematical measures. Symmetry-resolved entanglement entropy is one such measure. Their study focuses on special quantum states which are excited with respect to a ground state. The research shows how the entanglement amongst quantum particles can be measured and assesses the contribution to the entanglement of quasiparticle excitations, particularly in the presence of additional symmetries.
Dr Tsun-Kong Sham – Dr Jiatang Chen – Dr Zou Finfrock – Dr Zhiqiang Wang | X-Rays Shine Light on Fuel Cell Catalysts
Understanding the electronic behaviour of fuel cell catalysts can be difficult using standard experimental techniques, although this knowledge is critical to their fine-tuning and optimisation. Dr Jiatang Chen at the University of Western Ontario works with colleagues to use the cutting-edge valence-to-core X-ray emission spectroscopy method to determine the precise electronic effects of altering the amounts of platinum and nickel in platinum-nickel catalysts used in fuel cells. Their research demonstrates the potential application of this technique to analysing battery materials, catalysts, and even cancer drug molecules.
Dr Marcus Noack and Dr Mark Risser | Advancing Gaussian Processes: The Noack-Risser Method
Dr Marcus Noack and Dr Mark Risser, researchers at Lawrence Berkeley National Laboratory, have recently proposed a significant advancement in the area of machine learning and data science that promises significant computational improvements: the enhancement of exact Gaussian Processes for large datasets, significantly improving data analysis capabilities for samples even beyond 5 million data points.
Dr Gregory Duane | Predicting Climate Change with Supermodels
Our universe is comprised of fascinatingly complex systems. Systems such as the Earth’s climate can, at first glance, seem far too complex and chaotic to predict accurately. Dr Gregory Duane and his team at the University of Colorado have been developing complex computational models that can learn from past data, providing us with intriguing insights and more accurate predictions about the future.