Professor Christian Laforsch | Professor Andreas Greiner – Microplastics: Solutions for a Persistent Pollutant
Plastics have revolutionised human existence. Medicine, technology, agriculture and construction all rely on highly durable plastic materials. However, the enduring legacy of plastics extends far beyond our cities and towns. Everywhere we look, from the deepest parts of the oceans to alpine glaciers, we find tiny fragments called microplastics. Recently, the collaborative research centre, ‘CRC 1357 Microplastic’, at the University of Bayreuth was granted a second funding phase by the German Science Foundation, to continue their intensive research into microplastics. The CRC 1357 team studies the formation and behaviour of microplastics in the environment and their long-term effects on soils, plants, organisms, and ecosystem processes. Through their research, the University of Bayreuth will be able to contribute to ground-breaking recommendations for policy-makers, industry and society.
Plastics: A Double-Edged Sword
Look around your home, office, or town, and you’ll quickly find something made of plastic. These materials are ubiquitous for a reason; their reliable stability under various environmental conditions makes them highly effective materials in diverse industries, including medicine, construction, manufacturing, textiles, food packaging, and technology. Plus, they are lightweight and have lower production costs than other materials, such as glass or metal.
In the 1970s, commodity plastic use soared, with single-use, disposable items dominating most industries. Quickly, plastics overfilled landfills and littering made it hard to ignore the glaring effects of our plastic addiction. Now, we find plastic everywhere: in raindrops, on mountain tops, on the bottom of lakes and oceans, and even in our bodies.
Plastic production continues to increase rapidly, with global production currently at 400 million metric tons per year. A large portion of plastics are recycled or incinerated. However, another large portion ends up in landfills, easily leaking into the environment. Their durability means that most plastics that have ended up in the environment are still there, creating a whole suite of ecological and environmental issues that go far beyond the nasty aesthetics of littering. Once plastic is in the environment, it fragments because of solar radiation and mechanical, chemical or biological stress. The resulting small particles are called microplastics.
Specifically, the term microplastics refers to the fragments of plastics smaller than 5 mm. Microplastics exhibit different behaviours in the environment compared to the material they originated from. For example, one common type of microplastic is sloughed from car tires as they abrade against roads.
If current production continues, roughly 12 billion tons of plastic waste will accumulate in natural environments and landfills by 2050. Once these plastics enter the environment and become microplastics, they can have severe consequences for wildlife and ecosystems. Though their small size makes their impacts less visually obvious, microplastics can be easily ingested and enter terrestrial and aquatic food webs.
This ubiquitous contamination of the environment, and the associated risks to ecosystems and human health, have attracted much public attention. Yet, our knowledge of how microplastics form and migrate between ecosystems is still rudimentary. We also don’t understand how persistent microplastic pollution affects various organisms, including humans, in aquatic and terrestrial habitats and how these impacts alter essential ecosystem processes. Worryingly, many scientists believe that we are outside of the safe operating space, since the annual production of plastic is increasing at a pace that outstrips our global capacity for monitoring. This dangerous combination of ignorance and ubiquity caused the G7 summit to classify plastic pollution as a ‘top emerging global issue’.
However, solving the problem is not as simple as cutting ourselves off. Though our overproduction and reliance on plastics have caused various issues, plastics often play a central role in environmentally friendly actions. For example, the blades of wind turbines are typically made from lightweight epoxy plastic. Plus, without plastics, we wouldn’t have smartphones or even computers. Thus, research focused on microplastic pollution will need to be paired with innovative studies creating new types of plastics that have high stability yet are easier to recycle and are environmentally friendly.
Fortunately, some of the world’s top researchers have begun to tackle some of our pervasive knowledge gaps in microplastics, and are beginning to develop novel solutions. Led by Professor Christian Laforsch and Professor Andreas Greiner, the CRC 1357 Microplastics group at the University of Bayreuth in Germany stands out for its interdisciplinary approach to studying microplastic pollution.
Starting from model systems and moving towards more complex and realistic systems, researchers from CRC 1357 investigate the physical, chemical, and biological processes that microplastics experience in the environment. Their interdisciplinary expertise ranges from understanding how plastic affects single cells to tracking how these particles migrate through global ecosystems. The researchers use their comprehensive knowledge of the entire lifecycle of a microplastic to develop novel materials that help us wean from our plastic addiction.
Biological Interactions with Microplastic
Given their small size, many organisms – including humans – unwittingly ingest microplastics. Once microplastics are ingested, they come in contact with cells and tissues, causing inflammation, cell death and can move into the circulatory system.
The chemical and physical properties of microplastic particles play an important role in their interaction with cells and tissues. For example, one study by CRC 1357 PhD students Anja Ramsperger, Julia Jasinski and Matthias Völkl showed that supposedly identical microplastic particles from different commercial manufacturers were fundamentally different in their properties, leading to varying cell responses. Hence, the team showed that the characteristics of plastic particles have a crucial impact on the hazard assessment of microplastics.
As well as their varying characteristics, other factors can alter the biological interactions with microplastic. For example, microplastic particles exposed to the environment can become coated with biomolecules – forming a so-called eco-corona. With this knowledge, Anja Ramsperger wondered whether such a coating would affect the cellular internalisation compared to pristine microplastic particles.
Together with an interdisciplinary team within CRC 1357, she investigated whether macrophages would more easily internalise microplastic particles exposed to fresh or saltwater. Macrophages are some of the first responders– specialised cells that engulf foreign matter. Macrophage ingestion might be a key route for microplastics to get into tissues, where they may cause severe damage.
Their results showed that such exposed microplastic particles attached to and became internalised by the cells approximately ten times more often than pristine microplastic particles. Furthermore, when the team investigated the microplastics closer, they found that the particles exposed to fresh or saltwater had irregular surfaces, indicating that a coating of biomolecules had attached to the microplastic. In contrast, the pristine microplastic had plain surfaces.
The results of the CRC 1357 researchers demonstrate that the characteristics of plastics and environmental coatings play important roles in the interaction of microplastics with cells and tissues. Both findings are critical first steps for future studies predicting how ingested plastics might affect human health and wildlife.
Movement of Microplastics
Microplastics take diverse routes that transport them into ecosystems and remote habitats worldwide. To understand the distribution of microplastics, we need to map these transportation routes. Large bodies of water, such as lakes and oceans, are commonly considered sinks where microplastics can accumulate over time. On the other hand, atmospheric winds can transport and redistribute microplastics. Most researchers believe that the transportation of microplastics from bodies of water to the atmosphere is a vital migration pathway for microplastic particles. However, this pathway has largely been understudied.
CRC 1357 PhD student Mortiz Lehmann used high-end 3D models to investigate one potential pathway for microplastic transport between the atmosphere and aquatic systems: raindrops. When raindrops come in contact with a body of water, the impact site shoots upward at very high speeds and can eject more than a hundred droplets into the air. Because the surfaces of most bodies of water contain microplastics, the team wondered whether raindrop impacts could act as a possible pathway for microplastic transition from water to the atmosphere.
To test this idea, the researchers improved and optimised a state-of-the-art simulation tool to model fluid flow. They simulated more than 1600 impacts of raindrops at different angles, drop diameters, and randomly placed microplastics in various positions on the water surface. They paired these simulations with laboratory experiments demonstrating microplastic particles in splash droplets.
Based on the team’s observations, typical precipitation and wind data, they estimated the number of microplastic particles that transitioned from the global oceans into the atmosphere annually due to raindrop impacts. The final numbers were staggering: up to a hundred trillion particles transition from global oceans into the atmosphere every year.
This study conclusively showed that microplastic particle transport occurs across the water-air interface during raindrop impacts on the sea surface. In addition, a significant proportion of these microplastic particles are probably quickly removed from the atmosphere by falling raindrops. However, changes in the particle surface may occur due to the forces involved in the transition process. Nevertheless, the study shows that interface transitions and atmospheric transportation have a strong potential to redistribute large amounts of microplastics.
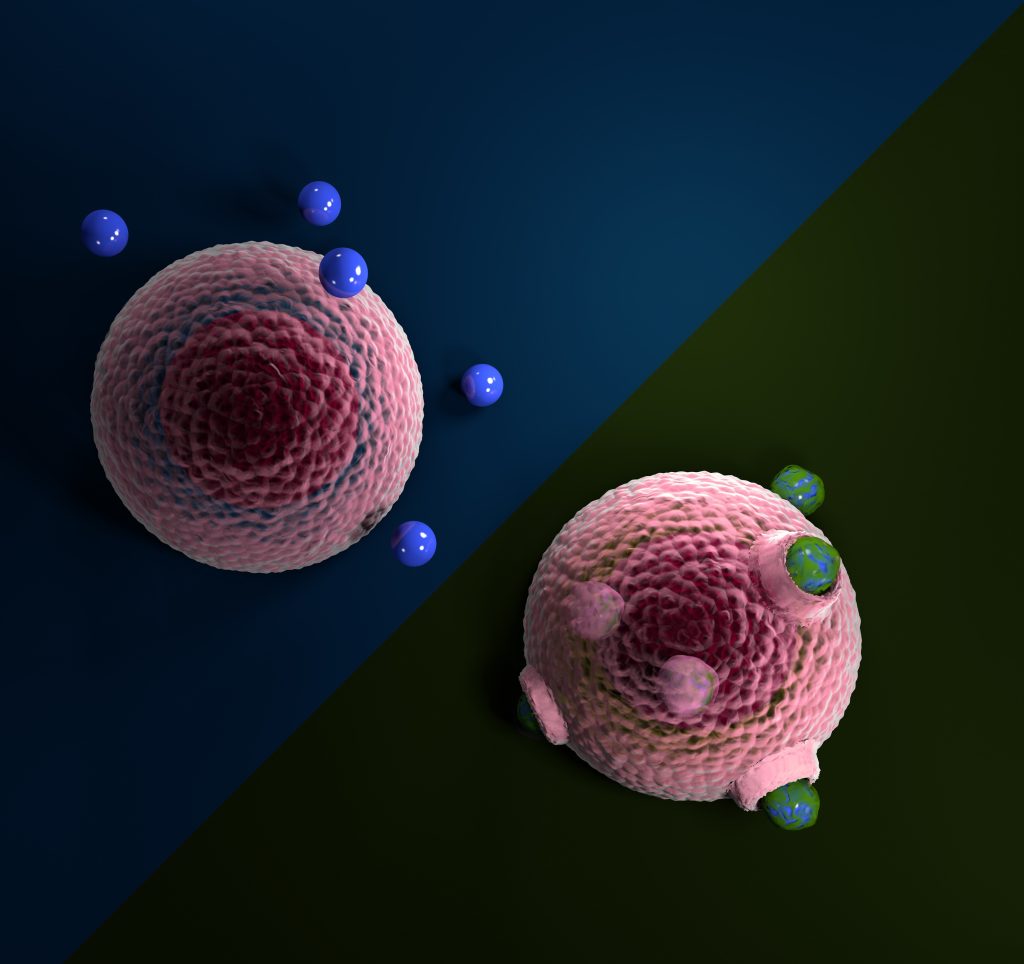
Macrophages ingesting microplastic particles coated by an ecocorona (blue with green coating), whereas plain plastic particles (clear blue) are refused.
Stress Accelerates Microplastic Degradation
Currently, we only have a basic understanding of how larger pieces of plastic degrade into smaller microplastic particles. Furthermore, we still lack detailed mechanistic knowledge of the processes driving plastic degradation, especially in relation to fragmentation under various environmental conditions. However, without clearly understanding this, we cannot create reliable risk assessments for the better storage and disposal of plastics. This further hampers the development of new environmentally friendly polymers.
Alongside an interdisciplinary team, CRC 1357 PhD students Nora Meides, Teresa Menzel, and Anika Mauel used accelerated weathering to simulate the environmental degradation of three polymers commonly found as waste in the environment: polystyrene (PS), low-density polyethylene (LDPE), and polypropylene (PE). They used various analytical techniques combined with simulations to mimic this long-term degradation on a reasonable time scale. The main mechanisms were degradation by UV radiation and mechanical forces through natural stress, such as the movement of seawater. Their findings verified that degradation from large plastic pieces into microplastic occurs over multiple stages, resulting in a multitude of tiny fragments.
In the first stage, the surface layer of the plastic is broken down by solar radiation and reactions with oxygen. Then in stage two, microcracks are formed, weakening the polymer structure and accelerating its degradation. During fragmentation, the molecular chains of the polymer become shorter and more branched. In this way, one polystyrene particle generates 500 small daughter particles in just 1.5 years of outdoor weathering. In one of their papers, the team provides details on both stages that can be used to predict the degradation of other common plastics. Notably, the researchers show that the speed and extent of degradation largely depend on the intensity of the applied stresses. For example, plastics exposed to solar radiation will degrade much more slowly when not additionally exposed to mechanical stress. Compared to LDPE and polypropylene, this process is relatively slow for polystyrene.
The fragmentation of LDPE and polypropylene involves a third step not reached by polystyrene within the observed time frame. Owing to a more considerable fragmentation, leading to the formation of smaller particles, LDPE and polypropylene tend to aggregate due to the changing surface characteristics. These secondary particles rarely occur as individuals in the environment, as they adhere to other particles.
A second profound difference is the speed of the break-up processes in stage 2. The disintegration of LDPE and polypropylene proceeds via surface fragmentation, significantly increasing the particle production rate. For example, from one polypropylene particle, 100,000 small daughter particles are generated in 2 years, and 14,000 small daughter particles are released from one LDPE particle within 1.5 years of outdoor weathering in Central Europe. These fragmentation rates are several orders of magnitude higher than those observed for polystyrene.
Although the studies used laboratory-controlled weathering conditions, the team’s results can be extrapolated to better understand microplastic degradation in natural environments. Their results, specifically those relating to polypropylene degradation, highlight the immense secondary pollution resulting from inadequate waste disposal. Other factors beyond the environmental stresses selected for these studies, such as temperature, microbes, oxygen, and salinity, will also affect microplastic degradation and the lifetime of microplastics in the environment. In contrast, additives such as UV stabilisers will extend the lifetime of polymers.
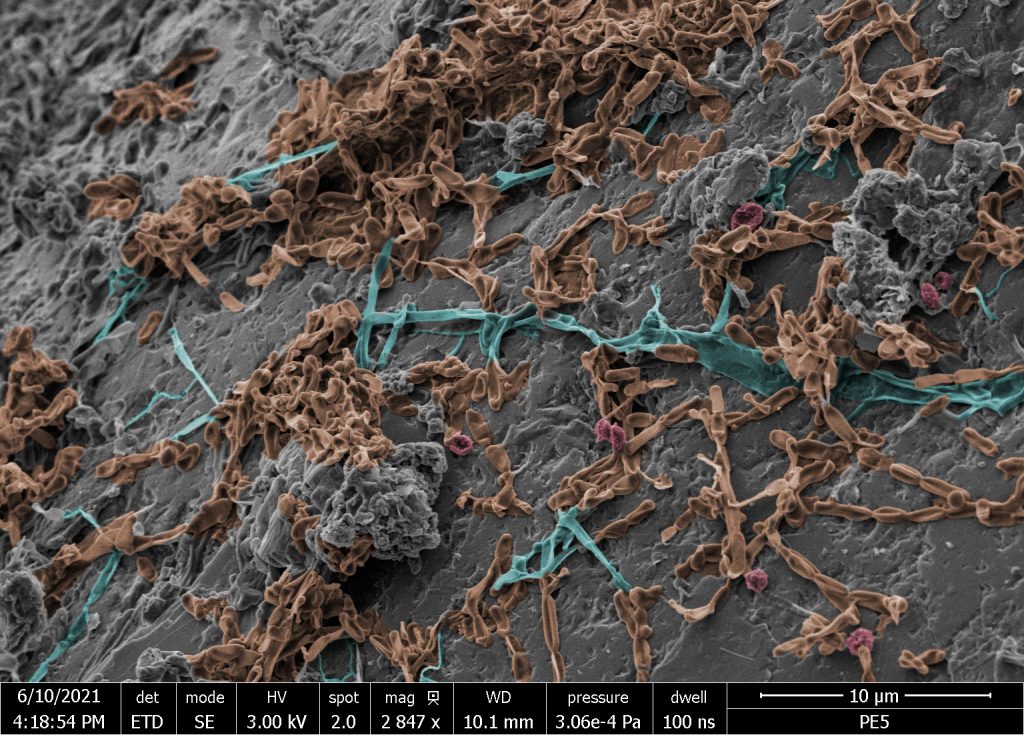
‘Fungal Plastisphere Jungle’ on spherical microplastic buried for 5 months in soil of a landfill in Germany (blue: hyphae, orange: blastospores and pseudohyphae (yeasts), red: conidia/spores). The image was produced by Gerasimos Gkoutselis PhD within the CRC 1357 Microplastic and Ulrich Mansfeld from the Keylab Electron Microscopy of the University of Bayreuth.
The Plastisphere
A poorly understood aspect of plastic pollution is the interaction between plastics and microbes. The surface of plastic waste provides an ideal environment for microbes to colonise, forming diverse communities called ‘biofilms’, otherwise known as the ‘plastisphere’. Often, microplastics attract microbial communities that are different from those found in the immediate surrounding environment. In this way, the plastisphere formed by microplastics represents a unique microhabitat, which can have unprecedented effects on local ecosystems. Moreover, as microplastics can influence the soil microbial community, they can strongly impact ecosystem services such as carbon sequestration.
The role of microplastics as a reservoir for harmful microbes has long been proposed, but has received comparatively little research attention. Many knowledge gaps exist concerning microbes and fungi that accumulate on plastics in terrestrial environments. PhD students Gerasimos Gkoutselis and Stephan Rohrbach, a mycologist and microbiologist working together within CRC 1357, provided the first in-depth insights into the development of fungal communities in the soil plastisphere. They also evaluated whether microplastics can harbour pathogenic fungi in terrestrial habitats. Specifically, they assessed plastisphere microbial communities in a German dump and fungal communities in the topsoil of five sites in Siaya, Western Kenya. Their sites included two landfills, a marketplace, a roadside, and a courtyard.
The team found rich fungal communities, showing that microplastics can be regarded as microhabitats for a diversity of fungi. The plastics only contained 76% of the total fungal species in the surrounding soil, suggesting that plastic selects for certain fungi, likely those with certain adhesive abilities, resulting in an uneven and artificial community.
Remarkably, many of the fungi the researchers found were pathogens of plants and animals, with the most dominant species being opportunistic human pathogens. In their survey, the team found the fungus Fusarium oxysporum, which causes severe eye infections in humans, and Rhodotorula mucilaginosa, a species that can thrive in the bloodstream and has been shown to cause serious blood infections in hospital patients. Furthermore, they found a selective accumulation of yeasts that cause invasive lung and brain infections.
This study is the first to demonstrate the direct impact of plastic pollution on the accumulation of soil-borne pathogens, with several ecological and epidemiological implications. Most notably, given that plastic is omnipresent worldwide, microplastics likely open new infection routes that increase disease risk for wildlife, livestock, and humans. Furthermore, microplastic can shape the microbial community of soil, and thus its capacity for necessary ecosystem services.
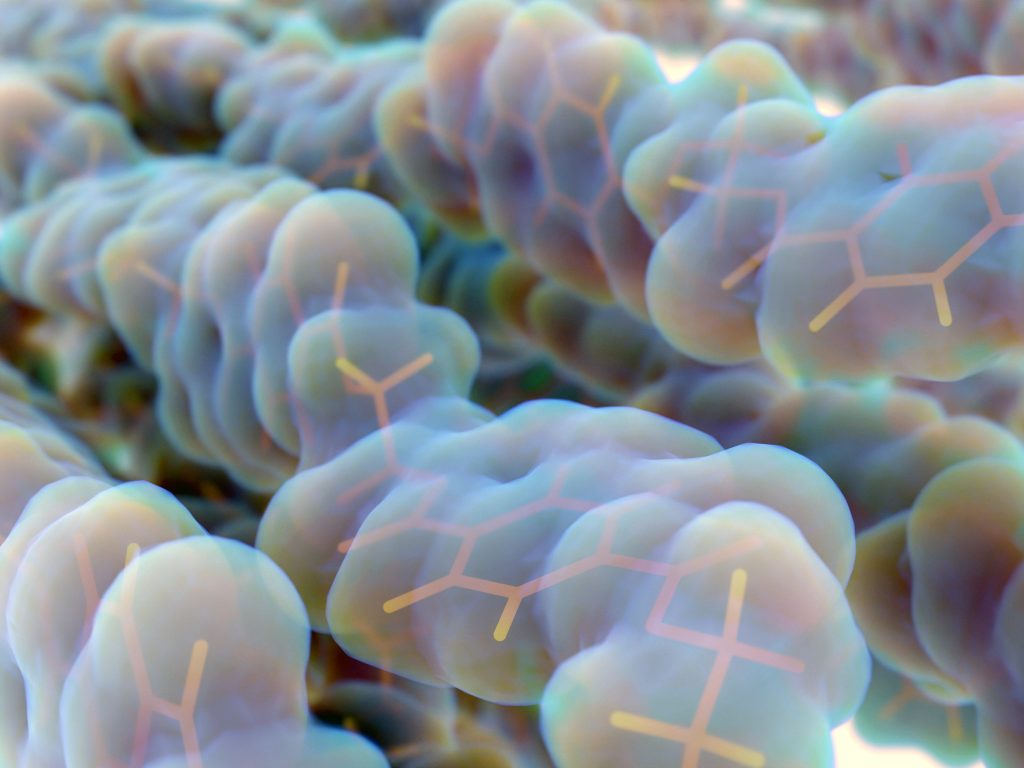
Biodegradable polymer molecules.
Solutions
Within CRC 1357, various projects are underway to create new types of materials that achieve the ideal balance between durability and environmental degradability.
In one project, CRC 1357 researchers and international colleagues considered whether natural tree gum waste could be modified to form stable, biodegradable materials. In its natural state, tree gum waste is weak and brittle. However, when the researchers chemically modified the material, the resulting material was not only considerably stronger but biodegraded within 28 days. The material also had excellent antibacterial properties – an essential feature that determines the shelf life of packaged goods. This novel research highlights that this material could be used in the environmental and food packaging industries.
Along with experimenting with natural waste products, CRC 1357 researchers have also conducted various studies in which they changed the chemical properties of many plastics, such that they degrade more quickly without leaving any persistent microplastics. Currently, biodegradable packaging conflicts with the need for high-performing packaging materials to reduce food waste, although they can help prevent plastic accumulation in our environment.
Combining biodegradable polymers with vermiculite nanosheets can accelerate the degradation rate and improve the barrier properties of the polymers. Using this approach, CRC 1357 PhD students Volodymyr Dudko and Renee Timmins created materials that are not only more degradable than existing biodegradable polymers, but also have similar durability and affordability to non-biodegradable plastics.
Finally, CRC 1357 PhD student Elmar Sehl created a new co-polyester platform, which provides an ideal balance between mechanical properties and fast hydrolysis. These materials also showed rapid enzymatic degradation. With PhD student Renee Timmins, he applied a thin nanocomposite coating to such a substrate, leading to a material with a drastically increased oxygen barrier, while maintaining the good mechanical properties and optimum composting time of less than eight weeks.
Moving Forward
In general, research on microplastics is still a growing field. Thanks to research done at CRC 1357, we understand that the complex effects of microplastics depend on the properties of the particles, their interactions with microorganisms, and how they move between ecosystems.
The urgency of the plastic crisis has also led to innovative experiments, with new products being developed that aim to strike the perfect balance between durability and sustainability. Collectively, our society faces an immediate challenge: coming to terms with the fact that we need plastics, while reducing our dependence on single-use plastics designed for convenience. Along with behavioural changes, we must invest in groups such as CRC 1357, whose interdisciplinary and thorough research is critical to improving how we deal with valuable plastic materials, towards a safer and more sustainable way of life.
As the group’s Spokesperson Professor Laforsch concludes: ‘Only through cooperation between the various scientific field is it possible to approach the challenges that this topic poses to science. Plastics are a valuable material, and our task is to enable a future-oriented and ecologically sustainable use.’
SHARE
DOWNLOAD E-BOOK
REFERENCE
https://doi.org/10.33548/SCIENTIA881
MEET THE RESEARCHERS
Professor Christian Laforsch
Spokesperson of CRC 1357 Microplastics
Department of Biology
University of Bayreuth
Bayreuth
Germany
Professor Christian Laforsch was awarded his PhD in Biology from Ludwig-Maximilians-Universität (LMU) Munich in 2003. Upon graduating, he worked at several prestigious universities, including the University of Leipzig, Lancaster University and LMU. In 2012, he started his current role as a Professor and Chair of Animal Ecology at the University of Bayreuth. Here, Professor Laforsch is the Spokesperson for the collaborative research centre, CRC 1357 Microplastics and speaker of the International Training Network LimnoPlast. His research focuses on aquatic ecology and evolutionary ecology, particularly how organisms adapt to a changing environment through phenotypic plasticity.
CONTACT
E: christian.laforsch@uni-bayreuth.de
Professor Andreas Greiner
Co-spokesperson of CRC 1357 Microplastics
Department of Biology
University of Bayreuth
Bayreuth
Germany
Professor Andreas Greiner was awarded his PhD in Chemistry from Philipps-University Marburg in 1988. Upon graduating, he worked at several prestigious universities, including the Johannes-Gutenberg-Universität Mainz, the Philipps-University Marburg, and the University of California Santa Barbara. In 2012, he started his current role as a Professor and Chair of Macromolecular Chemistry at the University of Bayreuth. Here, he is Co-spokeperson of the collaborative research centre, CRC 1357 Microplastics. Moreover, he is Co-founder and Editor-in-Chief of e-Polymers (De Gruyter) and Business Unit Manager at Neue Materialien Bayreuth GmbH.
CONTACT
E: greiner@uni-bayreuth.de
W: www.mcii.uni-bayreuth.de/en
W: www.mcii.uni-bayreuth.de/en/research/researchgreiner/index.html
FUNDING
Deutsche Forschungsgemeinschaft (DFG)
WEBSITE
https://www.sfb-mikroplastik.uni-bayreuth.de/en/index.html
REPUBLISH OUR ARTICLES
We encourage all formats of sharing and republishing of our articles. Whether you want to host on your website, publication or blog, we welcome this. Find out more
Creative Commons Licence (CC BY 4.0)
This work is licensed under a Creative Commons Attribution 4.0 International License.
What does this mean?
Share: You can copy and redistribute the material in any medium or format
Adapt: You can change, and build upon the material for any purpose, even commercially.
Credit: You must give appropriate credit, provide a link to the license, and indicate if changes were made.
SUBSCRIBE NOW
Follow Us
MORE ARTICLES YOU MAY LIKE
Dr Robert Larkin | Cultivating Change to Improve Soil Health and Increase Potato Yield
Environmental quality and food production are facing the pressing challenges of climate change and global population growth. Dr Robert Larkin from the United States Department of Agriculture-Agricultural Research Service (USDA-ARS) and a team of plant scientists developed and tested a range of crop management systems to help overcome these compounding challenges. Their work is improving soil health and increasing the yield of potato crops, contributing to the future food security of nations.
Dr Olalla Castro-Alvaredo | Measuring Entanglement: Symmetry-Resolved Entropy
Dr Olalla Castro-Alvaredo of the City University of London (UK) and her collaborators are advancing our understanding of an important phenomenon of quantum mechanical systems known as entanglement and, especially, its mathematical measures. Symmetry-resolved entanglement entropy is one such measure. Their study focuses on special quantum states which are excited with respect to a ground state. The research shows how the entanglement amongst quantum particles can be measured and assesses the contribution to the entanglement of quasiparticle excitations, particularly in the presence of additional symmetries.
Dr Arne Stensvand | Thermotherapy: Effective Disease and Pest Management Without Chemicals
Dr Arne Stensvand and his team at the Norwegian Institute of Bioeconomy Research are developing physical methods of pest reduction in plants. The team is specifically interested in strawberry plants, for which pest management is vital for crop success. They are pioneering thermotherapy as a heat treatment method to provide an environmentally effective and economically sound non-chemical approach to pest management.
Dr. Daniel Canfield | Reprioritising Pollution Reduction in Florida’s Lakes
Florida’s landscape is dotted with thousands of lakes that reflect regional geology, topography and anthropogenic activities. Phosphorus and nitrogen are critical nutrients for maintaining the wide range of biological production expressed across Florida, but excessive inputs of these nutrients due to past human activities impair many waters. There has been a long history of work aiming to address associated water quality pressures, and Dr. Daniel Canfield at the University of Florida has been at the centre of these efforts for over 40 years. Now, with the correction of point-source nutrient inputs, Dr. Canfield proposes that holistic lake management, including the integration of in-lake management strategies with a focus on organic sediment removal, should be much more prominent on the US government’s agenda to provide faster restoration of stakeholders’ lake usability.