Logan Kossel | John Pfotenhauer – Advancing Cryogenic Technologies Through Pulsating Heat Pipes
Comprising thin tubes that contain ultra-cold liquids and vapours, ‘cryogenic pulsating heat pipes’ can transport heat far more rapidly than even the most conductive metals. Logan Kossel and John Pfotenhauer at the University of Wisconsin-Madison are exploring the unique capabilities of this technology in unprecedented levels of detail. Through their research, they hope to boost the performance of pulsating heat pipes even further – potentially leading to new breakthroughs in many technologies that rely on cryogenic temperatures.
Heat Flow at Cryogenic Temperatures
The ability to manipulate heat flow is a crucial requirement in many cutting-edge technologies. For the newly-launched James Webb Space Telescope, for example, its detectors need to pick up the barely perceptible signals of galaxies occupying the most distant reaches of the observable universe. Unless these instruments are maintained at temperatures close to absolute zero (around −273°C), the faintest signals they detect would be drowned out by random fluctuations in detected heat.
To avoid this problem, any heat generated by the spacecraft needs to be transferred to an ultra-cold radiator, or ‘cryocooler’, where it can be transmitted out into space. However, in the shady environment of the James Webb Space Telescope’s sunshield – which blocks out almost all of the Sun’s radiation – there is very little difference in temperature between the telescope’s detectors and cryocoolers, making heat transfer especially challenging.
‘A temperature difference is fundamentally required to transfer heat,’ explains Dr Pfotenhauer, a Professor at the University of Wisconsin-Madison. ‘Ideally, however, we would like to transfer the heat with as little of a temperature difference as possible.’
This same problem is also relevant in technologies across the field of cryogenics, which all involve cooling fluids to temperatures close to absolute zero. Today, perhaps the most well-suited method for transporting heat within these ultra-cold systems is a device named the ‘pulsating heat pipe’.
The Heat Pipe Concept
In more traditional heat pipes, which connect warm heat sources with cooler heat sinks, the energy absorbed at the pipe’s warmer end is transferred to a liquid in contact with its inner surface. The liquid vaporises, and this vapour then travels to the colder end of the pipe, where it condenses, releasing the heat it absorbed as it evaporated. Finally, the liquid returns to the hotter end of the pipe through a wicking mechanism along the walls, and the cycle repeats.
In the early 1990s, researchers in Japan introduced a simpler design, featuring a snake-like arrangement of pipes without any internal wick. ‘The pulsating heat pipe comprises a capillary sized tube, extending back and forth between a heat source and a heat sink, and partially filled with some fluid,’ Dr Pfotenhauer describes. ‘Its most exciting feature is that it can transfer heat very effectively with very little temperature difference between the ends.’
Faster Heat Transfer
The pulsating heat pipe’s structure can essentially be broken down into three parts. Firstly, the colder end, or ‘condenser’, takes up the top 10% of the pipe’s structure. Here, the pipe repeatedly passes through the heat sink, condensing the vapour inside into a liquid. The opposite occurs at the hotter end, or ‘evaporator’ – which takes up the bottom 10% of the pipe. Finally, the ‘adiabatic’ region occupies the space between the sink and source, and must be traversed by fluid inside the pipe in order to transfer heat between both ends.
Because the pipe is so narrow, the surface tension force, being larger than gravitational force, prohibits the liquid from collecting at the lower end. Instead, the alternating liquid and vapor regions are maintained. ‘Vapor regions growing as they go through the evaporator and shrinking as they go through the condenser give rise to a pulsating motion of the fluid,’ explains Dr Pfotenhauer. ‘Small differences between the expansion and contraction forces, from one loop of the pipe to the next, causes the fluid to spontaneously move between both ends – either following a single direction, or pulsating back and forth as the vapor bubbles compress and expand.’
Comparing Performance
To understand just how effectively pulsating heat pipes can convey heat, Dr Pfotenhauer and his PhD student Logan Kossel compared their performance with the heat-conducting capabilities of pure metals. ‘Maximum values of thermal conductivity range between 2,000 and 3,000 watts per meter per kelvin for high-purity copper or aluminium,’ Dr Pfotenhauer recalls. ‘In contrast, values as large as 300,000 watts per meter per kelvin have been reported for helium pulsating heat pipes.’
The device’s unmatched ability to transfer heat is made possible by the unusual properties of the cryogenic fluids it contains. Helium, for example, is around 100 times less viscous in liquid than liquid water at room temperature. To assess the overall performance of pulsating heat pipes at cryogenic temperatures, it is crucial for researchers to gain a detailed understanding of these behaviours.
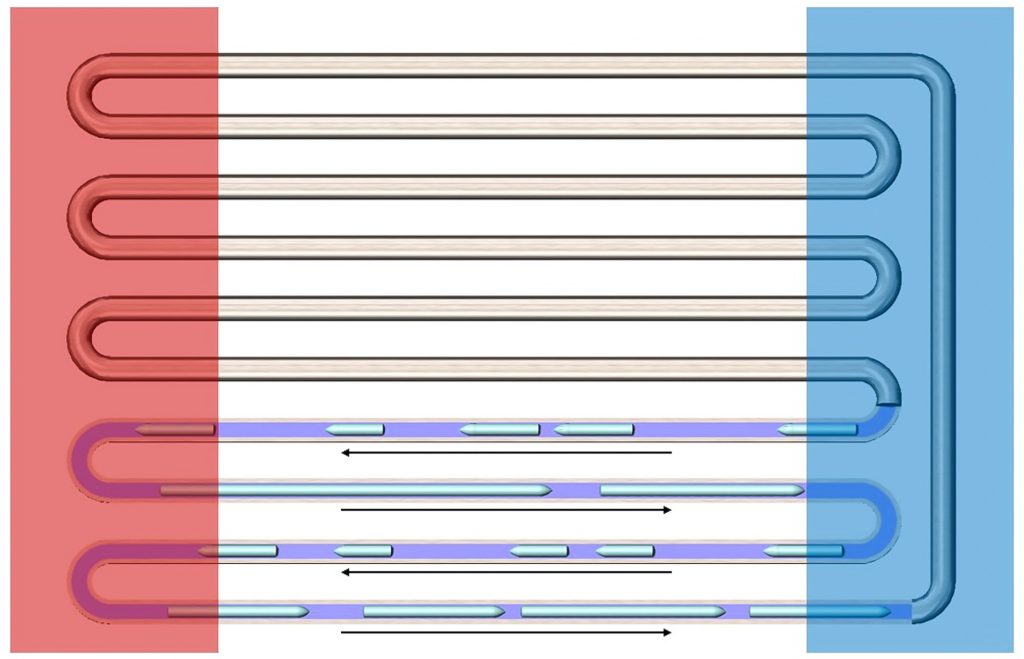
Cutaway view showing inside of the capillary tubes that make up a pulsating heat pipe.
Experiments and Models
Through their research, Kossel, Pfotenhauer and their colleagues have developed advanced techniques for gaining this level of understanding. ‘Since the properties of the fluids they contain are significantly different from fluids used for room-temperature pulsating heat pipes, we have initiated a variety of studies, both experimental and computer modelling, to characterise their behaviour,’ Dr Pfotenhauer explains.
The team’s modelling techniques are based on computational fluid dynamics – which can virtually recreate the complex physics underlying the motions of liquids and gases. By adjusting their models based on their experimental measurements, the researchers can ensure the best possible accuracy in their simulations – allowing them to visualise the motions of ultra-cold fluids in close detail.
‘The model enables us to “see” inside the cryogenic pulsating heat pipe, explore the fluid behaviour, and learn how the fluid motion influences the thermal behaviour,’ Dr Pfotenhauer continues. ‘As it flows through the condenser, the vapor breaks into smaller liquid regions and condenses as it is cooled.’
Surprises in Adiabatic Lengths
Out of the discoveries the team have made so far, one result stands out as particularly unusual. Where the length of the adiabatic region of pure metals has a direct effect on their rate of heat transfer, this doesn’t seem to be the case for pulsating heat pipes. ‘In high purity metals, the temperature difference would about three times larger for the 1 metre length than the 0.3 metre length,’ Dr Pfotenhauer describes. ‘Our data displayed the same temperature difference with an identical rate of heat transfer across pulsating heat pipes with a 1 metre and a 0.3 metre adiabatic length.’
This discovery could have important implications for the wide array of technologies that could benefit from pulsating heat pipes. In space telescopes, for example, detectors and cryocoolers are sometimes required to be far apart, separated by more than one metre. With pulsating heat pipes, heat can still be carried away from the telescope’s detectors just as rapidly as if they were placed in close proximity to the cryocooler. A large separation between the detector and the cryocooler is often necessary to avoid degrading the detector’s sensitivity from the cryocooler’s vibration.
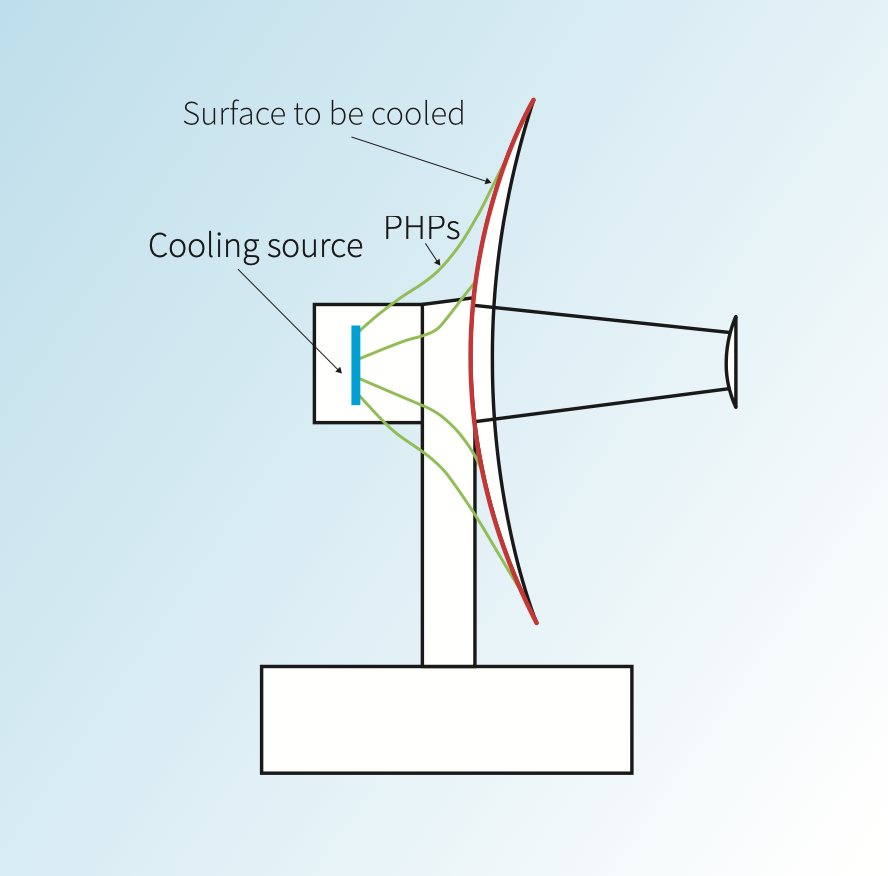
Cryogenic pulsating heat pipe extending from the cryocooler on the left to the detection surface of a space telescope on the right.
Examining Influential Factors
In their current research, Kossel, Pfotenhauer and their colleagues are continuing to explore many different factors that affect the performance of pulsating heat pipes. Their investigation can be divided into three broad areas. Firstly, by studying the geometry of the pipes, they hope to determine how long their adiabatic regions can become before their rate of heat transfer begins to diminish.
Secondly, the team is examining the factors that can be directly altered by the operators of cryogenic systems – including whether the pipes are oriented horizontally or vertically, and the proportion of their volume that is filled with fluid. Finally, the researchers are studying the properties of the fluid itself – including how the choice of helium, nitrogen, or hydrogen affects the device’s performance.
Pushing Cryogenic Technologies Further
By improving the ability to rapidly transport heat over large distances at ultra-cold temperatures, the researchers hope that their new insights could inspire breakthroughs across a wide array of cryogenic technologies. Their work could provide important guidance for researchers and engineers designing future generations of space telescopes – which may pick out the signatures of distant galaxies at even higher resolutions than the James Webb Space Telescope.
Elsewhere, more advanced approaches to pulsating heat pipe operation could lead to better designs for propellant holding tanks, which store fuels such as liquid hydrogen at ultra-cold temperatures. One hope is that the pulsating heat pipes will help to maintain a uniform temperature in the holding tanks and thereby avoid unwanted boil-off.
Even further, the team’s results could lead to new advances in superconductors, which offer zero resistance to electrical currents flowing through them. These materials are already being extensively investigated for their performance as highly compact magnets; but if pulsating heat pipes could be used to better maintain their ultra-low temperatures, Kossel, Pfotenhauer and their colleagues hope that their use could be expanded further.
If achieved, superconducting magnets may soon become widespread across numerous applications – ranging from MRI machines that produce high-resolution medical images, to nuclear fusion reactors that provide us with a clean and practically limitless source of energy.
SHARE
DOWNLOAD E-BOOK
REFERENCE
https://doi.org/10.33548/SCIENTIA857
MEET THE RESEARCHER
Logan M. Kossel
Cryogenics Engineering Lab
University of Wisconsin – Madison
Madison, WI
USA
Logan Kossel is a currently completing his PhD in Mechanical Engineering at UW-Madison’s Cryogenics Engineering Lab. He has also worked as a Visiting Technologist at both the NASA Glenn Research Center, and the NASA Jet Propulsion Laboratory. His thesis is titled ‘Low Mass Long Distance Effective Heat Transfer via Cryogenic Helium Pulsating Heat Pipes,’ and is funded by the NASA NSTGRO Graduate Fellowship. Kossel’s main research interests include cryogenic experiments and technology, and heat pipe technology.
CONTACT
E: lkossel@wisc.edu
W: https://www.linkedin.com/in/logankossel/
Dr John M. Pfotenhauer
Department of Mechanical Engineering
University of Wisconsin – Madison
Madison, WI
USA
Dr John Pfotenhauer achieved a PhD in Physics at the University of Oregon in 1984. He has worked as a Professor in UW-Madison’s Departments of Mechanical Engineering and Engineering Physics since 1993, and has also taken up guest positions at a variety of institutions across the US and China: including Oak Ridge National Laboratory, and the Chinese Academy of Sciences. Through his widely-varied research interests, he explores topics including the development and application of refrigeration cycles, and the use of cryogenics to capture pollutants from the waste products of coal-fired power plants.
CONTACT
E: pfot@engr.wisc.edu
W: https://directory.engr.wisc.edu/me/Faculty/Pfotenhauer_John/
KEY COLLABORATORS
Franklin K. Miller, UW Madison
Luis Diego Fonseca Flores, UW Madison (now at NASA Jet Propulsion Laboratory)
Bryant Mueller, UW Madison (now at NASA Jet Propulsion Laboratory)
Chen Xu, UW Madison (now at Modine Manufacturing Company)
Zhiyi Jiang, UW Madison
Mark Derakhshan, Sumitomo Cryogenics of America
Ben Gronemeyer, Sumitomo Cryogenics of America
Stephen Dunn, Sumitomo Cryogenics of America
Tian Lei, Sumitomo Cryogenics of America
Zhihua Gan, Zhejiang University
Xiao Sun, Zhejiang University (now at Midea Group)
FUNDING
GE Global Research Center: 2007–2010
Costa Rican student fellowship programs MICITT and CONICIT (Fonseca): 2010–2014
Sumitomo Cryogenics of America: 2015–2022
US Department of Energy (award # DE-SC-0019920): 2019–2020
China Scholarship Council (Sun): 2019–2020
NASA-NSTGRO (Kossel) NASA Grant Number 80NSSC20K1177: 2020–2024
REPUBLISH OUR ARTICLES
We encourage all formats of sharing and republishing of our articles. Whether you want to host on your website, publication or blog, we welcome this. Find out more
Creative Commons Licence (CC BY 4.0)
This work is licensed under a Creative Commons Attribution 4.0 International License.
What does this mean?
Share: You can copy and redistribute the material in any medium or format
Adapt: You can change, and build upon the material for any purpose, even commercially.
Credit: You must give appropriate credit, provide a link to the license, and indicate if changes were made.
SUBSCRIBE NOW
Follow Us
MORE ARTICLES YOU MAY LIKE
Professor Giorgio Buttazzo | Artificial Intelligence and a Crossroads for Humanity
Where do we stand with artificial intelligence? Might machines take over our jobs? Can machines become conscious? Might we be harmed by robots? What is the future of humanity? Professor Giorgio Buttazzo of Scuola Superiore Sant’Anna is an expert in artificial intelligence and neural networks. In a recent publication, he provides considered insights into some of the most pressing questions surrounding artificial intelligence and humanity.
Professor Martin Trefzer | Bridging Nature and Artificial Intelligence for Smart Electronics Technology
The ever-developing world of artificial intelligence (AI) stands at the tip of a transformative breakthrough. Professor Martin Trefzer from the University of York and Professor Jim Harkin from Ulster University have introduced a revolutionary approach to neural network design. They work on an electronic system based on AI that forms the basis of the cross-disciplinary project called Nervous Systems, which aims to build electronic neuromorphic devices with an artificial intelligence system mirroring the adaptability and responsiveness of biological neural systems.
Dr Jon Tore Lieng | Dynamically Installed Anchors for Floating Offshore Turbines
Effectively harnessing offshore wind presents a valuable opportunity to increase energy supplies. Floating wind turbines present several advantages over traditional fixed turbines in more shallow waters. Dr Jon Tore Lieng from Deep Sea Anchors and colleagues have developed a type of dynamically installed anchor to hold the structures in place while reducing both the costs and complexity associated with installation where cohesive seabed sediments are realised.
Dr Sébastien Weber | PyMoDAQ: Navigating the Future of Data Acquisition
In an era where data is paramount, Dr Sébastien Weber and his team at CNRS, the French National Centre for Scientific Research, are changing the landscape for scientists and engineers with PyMoDAQ, an open-source data acquisition software. Their revolutionary tool stands out for its accessibility, versatility, and the thriving community it fosters.