Dr Fiona Darbyshire | Ear to the Ground: How Earthquakes Reveal Earth’s Ancient History
Earthquakes are one of the more destructive phenomena we encounter on Earth. However, the seismic waves that earthquakes send travelling through the Earth are powerful tools to investigate the Earth’s crust and mantle. Through a series of seismic recording stations in Canada and northern USA, Dr Fiona Darbyshire at the Université du Québec à Montréal is doing exactly that. By listening to the seismic waves emitted from earthquakes, she and her team can determine the current composition of the crust and upper mantle, while also revealing tantalising clues about the formation and evolution of tectonic plates over the planet’s long history.
Exploring the Lithosphere
On time-scales of millions of years, Earth’s crust is constantly moving and changing. Tectonic plates collide, causing mountain ranges to rise and rifts to deepen, and transforming the face of the planet. This movement is not only incredibly gradual, but is also concealed several thousand metres beneath our feet.
At this depth, it is impossible to simply dig a hole to investigate, so researchers need another method to reveal the dynamic processes within Earth’s lithosphere – the rigid layer that includes the crust and the upper portion of the mantle.
This is where earthquakes can provide a wealth of information. When an earthquake occurs, it transmits seismic waves from its origin through the interior of the Earth. As it travels through the Earth, the speed and direction of a seismic wave is affected by the density, depth, temperature and composition of the rock it passes through.
By building a series of seismograph stations, scientists can detect seismic waves caused by earthquakes. By comparing the data gathered from earthquakes at many ‘listening’ stations at diverse locations, they can build a picture that reveals the thickness, temperature and composition of Earth’s lithosphere, as well as the interaction between this solid outer-most layer and the flowing mantle beneath.
Dr Fiona Darbyshire at the Université du Québec à Montréal has used a suite of these listening stations across Canada, Greenland and north-eastern USA to study seismic waves. Her investigations have revealed critical insights into Earth’s lithosphere, and its evolution over billions of years.
‘I am a structural seismologist who uses the seismic waves from large global earthquakes, recorded by seismograph stations, to study the crust and upper mantle beneath these regions,’ says Dr Darbyshire. ‘Each large earthquake provides several complementary sources of information in the form of body waves and surface waves, all of which can be analysed in different ways to explore different aspects of Earth’s structure.’
Subsurface Observations
By listening to the rumbles of seismic activity, Dr Darbyshire and her colleagues build detailed maps of the geological features that lie deep underground.
The process of building a complete picture of these geological formations is a lengthy one, starting with the installation of a network of tens-to-hundreds of seismic listening stations. These stations are placed in a large array, often in remote locations where little data has previously been collected. Much of Dr Darbyshire’s work has involved installing and taking data from an array spanning more than 800 kilometres through Canada and the USA.
‘My collaborators and I have been actively involved in installing seismograph stations in areas of eastern and northern Canada and the northern USA that have suffered from a lack of adequate data coverage in the past,’ she explains. ‘These include distributed long-term installations as well as denser networks that target areas of particular scientific interest.’
Once installed, these arrays of seismograph stations are in operation for years at a time, detecting everything from large earthquakes to faint seismic rumbles. At the end of the data collection period, Dr Darbyshire and her team have the daunting task of processing all the data produced.
They use these data to determine the speed and direction of seismic waves as they travel through the Earth. From this, they can determine the subsurface structure of the Earth and reveal geological formations that are not observable at the surface. The waves caused by a seismic event will travel through the Earth with no major change in speed or direction until they meet an anomaly or boundary.
One such boundary is where the crust meets the mantle, called the Moho. By measuring the seismic waves bouncing off this boundary, Dr Darbyshire and her team can create a so-called Moho depth map.
Other features that will affect the passage of seismic waves include orogens, where the crust crumples and uplifts to form mountain ranges, and cratons, which are the remnants of ancient continental plates. By painstakingly going through all the data generated by the networks of seismograph stations, Dr Darbyshire identifies the remains of ancient tectonic collisions and interactions, giving us unique insight into Earth’s distant past.
‘Wave speed models reveal imprints of previous tectonic processes such as rifting and mountain-building events, or large-scale deformation,’ explains Dr Darbyshire. ‘I therefore use the models in conjunction with surface geology and tectonic reconstructions to understand the history of formation and evolution of the continental lithosphere over its multi-billion-year lifetime. In addition, some of the models can provide information about the interaction between the plate and the actively convecting mantle beneath, as well as the patterns of this mantle flow.’
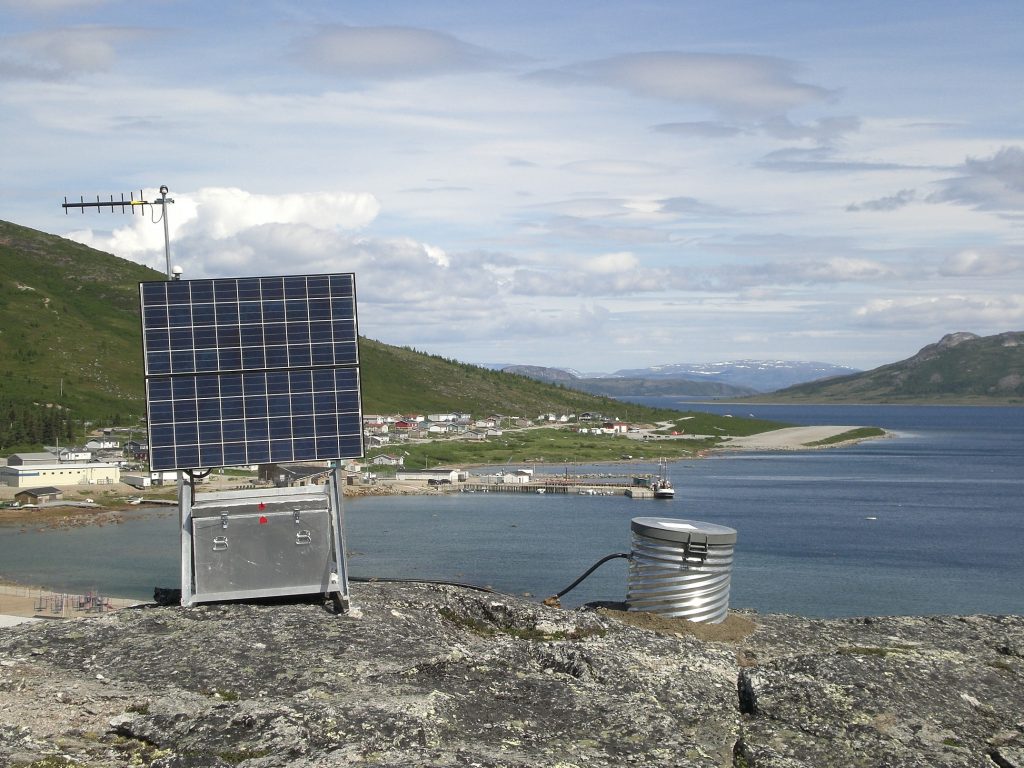
Seismograph station installed by Dr Darbyshire’s team in Nain, Canada, in 2011.
Past and Present Collide
One of the key questions facing Dr Darbyshire and her colleagues is in understanding the formation of the continents we see today. ‘There is still a great deal of debate regarding the formation and evolution of continental lithosphere,’ says Dr Darbyshire. ‘One of the biggest questions is whether “modern-style” plate tectonic processes have always existed on Earth and, if not, when did plate tectonics begin?’
By using the data collected by their seismograph stations, Dr Darbyshire and her team have been revealing insights into how the continents formed in the distant past. Specifically, their research aims to provide a better understanding of the geological history of the North American continent.
The North American continent is of special interest to geologists as some of its geological formations have been around for almost 4 billion years. As Earth is estimated to be approximately 4.5 billion years old, this means that these features must have formed very early on in the planet’s history. Such geological features therefore presented an incredible opportunity for Dr Darbyshire and her team to investigate the processes that drove the current arrangement of Earth’s continents, and how modern-day tectonic behaviour differs from its ancient form.
Features under the Appalachian Mountains in the north-eastern USA formed during our current geological eon, known as the Phanerozoic eon, which began around 538 million years ago. Nearby, the Laurentides territory of Québec hosts the Grenville Orogen, where landmasses collided and uplifted to form mountains about 1 billion years ago.
Further to the north under north-eastern Québec and Labrador, there is a series of cratons. These stable sections of the lithosphere formed in the Archean eon over 3 billion years ago, and are often found in the interiors of today’s continental plates. As they have survived for billions of years, despite being subjected to movement and collisions, they provide a snapshot of the very distant past.
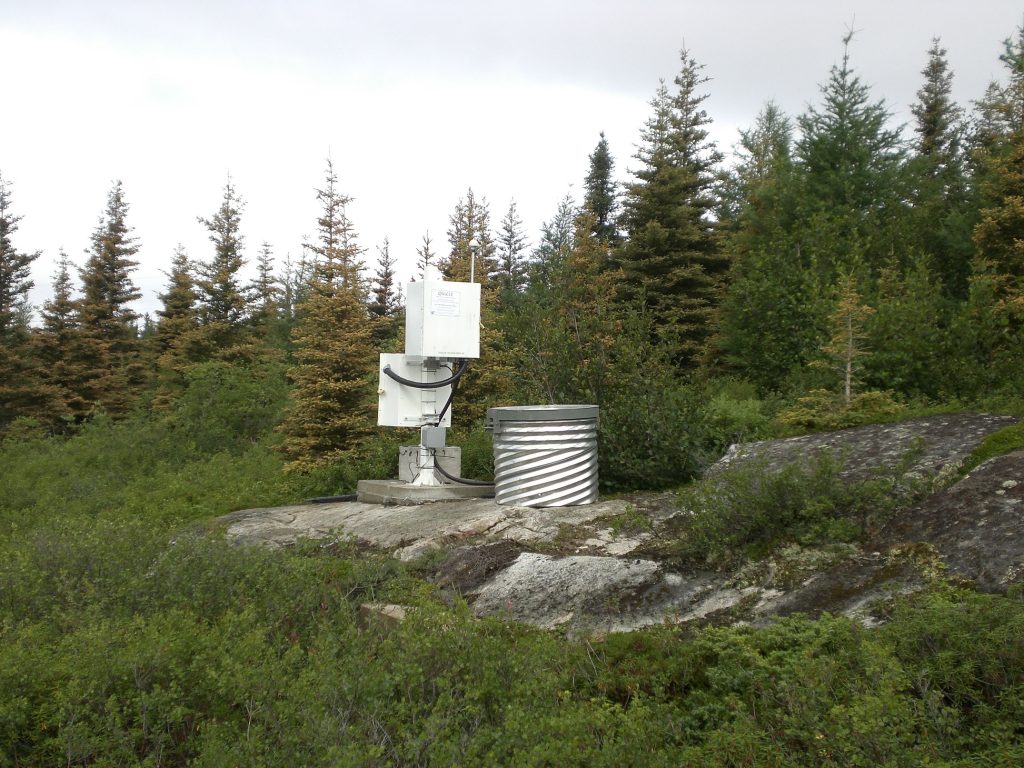
Seismograph station installed by Dr Darbyshire’s team in Kujjuarapik, Canada, in 2011.
These geological features have provided multiple new discoveries for Dr Darbyshire and her colleagues. For instance, Archean cratons found in the Churchill and Superior geological provinces in Canada are much more similar in composition and structure to each other than previously realised. Dr Darbyshire realised that any variations in the structures of these ancient continental cores were instead caused by tectonic events long after their formation.
‘We have shown that cratons are much more heterogeneous than previously realised, and the systematics in their variations can, in many cases, be correlated to their long-term tectonic evolution,’ says Dr Darbyshire.
Additionally, Dr Darbyshire and her team have gathered important data on the Trans-Hudson and Grenville Orogens, which formed vast mountain ranges between one and two billion years ago. As mentioned, orogens are regions where tectonic plates collide and uplift.
Interestingly, the ancient Trans-Hudson and Grenville Orogens bear a striking resemblance to the orogen that formed – and continues to form – the Himalayas. The researchers revealed very similar patterns of crustal thickness and composition between these ancient and active orogens. The similarities between the Himalayan-Karakoram-Tibetan Orogen and the Trans-Hudson and Grenville Orogens suggest that plate tectonic processes are the same across the world, and have also remained relatively unchanged for the last two billion years.
‘[This work provides] fundamental new constraints that contribute to the view that both orogens were similar in scale and nature to the present-day Himalayan-Karakoram-Tibetan Orogen and that modern-style plate tectonics was already in operation on the Earth by the early Proterozoic,’ explains Dr Darbyshire.
Her team’s research has greatly advanced our understanding of Earth’s lithosphere, particularly in regions that had not previously been studied in detail. ‘We are starting to understand more about the long and complex history of how the North American continent was built, and how it has evolved over a time period spanning about three-quarters of the Earth’s history,’ concludes Dr Darbyshire.
SHARE
DOWNLOAD E-BOOK
REFERENCE
https://doi.org/10.33548/SCIENTIA856
MEET THE RESEARCHER
Dr Fiona Darbyshire
Sciences de la Terre et de l’atmosphère
Université du Québec à Montréal
Montréal, Québec
Canada
Dr Fiona Darbyshire achieved her PhD at Cambridge University in 2000, for a project investigating the Earth’s crust in Iceland. Currently she is a full-time Professor at the Université du Québec à Montréal, and also holds an adjunct professorship at the University of Manitoba. Dr Darbyshire’s research has provided deep insights into Earth’s interior, and its evolution over the last three billion years. Over the course of her career, she has been granted with many honours, including being recognised with the Canada Research Chair title and a Canadian Geophysical Union Meritorious Service Award. Dr Darbyshire was also made an Ambassadeur for her work in organising the 27th IUGG General Assembly at the Palais des Congrès de Montréal.
CONTACT
E: darbyshire.fiona_ann@uqam.ca
W: https://www.geotop.ca/fr/membres/reguliers/F_Darbyshire
KEY COLLABORATORS
Andrew Frederiksen – University of Manitoba, Canada
Andrew Schaeffer, Riddhi Dave – Natural Resources Canada
Ian Bastow – Imperial College London, UK
Vadim Levin – Rutgers University, USA
Bill Menke – Lamont-Doherty Earth Observatory, USA
Suzan van der Lee, Seth Stein, Donna Jurdy – Northwestern University, USA
Douglas Wiens, Michael Wysession – Washington University at St. Louis, USA
Justin Revenaugh – University of Minnesota, USA
Randell Stephenson – University of Aberdeen, UK
Juan Carlos Afonso – Macquarie University, Australia & University of Twente, Netherlands
Trine Dahl-Jensen, Tine Larsen, Peter Voss – Geological Survey of Denmark and Greenland
Khaled Ali, Andrew Macdonald – De Beers
FUNDING
Natural Sciences and Engineering Research Council of Canada (NSERC)
Fonds de Recherche du Québec – Nature et technologies (FRQNT)
Canada Foundation for Innovation (CFI)
Natural Resources Canada GEM GeoNorth programme
De Beers Canada
US National Science Foundation
FURTHER READING
F Vervaet, F Darbyshire, Crustal structure around the margins of the eastern Superior craton, Canada, from receiver function analysis; Precambrian Research, 2022, 368, 106506.
O Bagherpur Mojaver, F Darbyshire, Crustal Structure Beneath the Northern Appalachians and the
Eastern Grenville Province, JGR Solid Earth, 2021, 127, e2021JB023246.
FA Darbyshire, ID Bastow, L Petrescu, A Gilligan, DA Thompson, A tale of two orogens: Crustal processes in the Proterozoic Trans-Hudson and Grenville Orogens, eastern Canada, Tectonics, 2017, 36, 1633-1659.
L Petrescu, ID Bastow, FA Darbyshire, A Gilligan, T Bodin, W Menke, and V Levin, Three billion years of crustal evolution in eastern Canada: Constraints from receiver functions, JGR Solid Earth, 2016, 121, 788-811.
REPUBLISH OUR ARTICLES
We encourage all formats of sharing and republishing of our articles. Whether you want to host on your website, publication or blog, we welcome this. Find out more
Creative Commons Licence (CC BY 4.0)
This work is licensed under a Creative Commons Attribution 4.0 International License.
What does this mean?
Share: You can copy and redistribute the material in any medium or format
Adapt: You can change, and build upon the material for any purpose, even commercially.
Credit: You must give appropriate credit, provide a link to the license, and indicate if changes were made.
SUBSCRIBE NOW
Follow Us
MORE ARTICLES YOU MAY LIKE
Dr Timothy Beers | Mapping the Galaxy’s Stellar Populations Using Large Photometric and Astrometric Surveys
Astronomers often use spectroscopic (electromagnetic radiation) data and astrometric (motion and positional) data to develop working models describing our Galaxy. Dr Timothy Beers from the University of Notre Dame and his collaborators in Korea and China combined large photometric (visible light) surveys and astrometric data to create multidimensional maps of a large part of the Galaxy. By highlighting significant inhomogeneities in stellar-chemical compositions, motions, and spatial distributions, Dr Beers and his colleagues provide valuable insights into how we can advance our understanding of the formation and evolution of our Galaxy.
Professor Gary Yohe | Navigating Climate Change: The Impactful Contributions of Gary Yohe
Professor Gary Yohe is a distinguished environmental economist whose work has been pivotal in shaping our understanding of climate change impacts, adaptation strategies, and policy frameworks. His interdisciplinary approach combines economics with environmental science, offering nuanced insights into global warming and its multifaceted impacts on natural and human systems. Professor Yohe equips us with the knowledge and strategies needed to navigate the complex and pressing challenges posed by climate change.
Dr Sebastian Fraune | Microbiota: Fast-tracking Adaptation to Rapidly Changing Environments
As climate change continues at an unprecedented pace, the processes of natural selection and genetic mutation can no longer fully explain how some organisms adapt to their rapidly changing environments. Dr Sebastian Fraune from Heinrich-Heine University and an international team of researchers are the first to demonstrate a causal relationship between changes in the microbiome and changes in thermal tolerance. They propose that microbiota-mediated transgenerational acclimatisation can account for how animals adapt to their environments in much shorter periods of time than classical theory would predict.
Dr Attila Borovics | Mitigating Climate Change: The ForestLab Project
Our forests provide important recreational, social, ecological and economic functions. The ForestLab project, led by Dr Attila Borovics at the University of Sopron in Hungary, has been set up to simultaneously protect and utilise this pre-cious resource. Their recent findings point to the importance of addressing ‘old wood’, that is, unused wood stock that currently exists in Hungarian forests and adopting technologies for forest management in the near future.