Professor Sergey Kravchenko – Professor Alexander Shashkin | Understanding Electron Behaviour in Solid State Physics
Professor Sergey Kravchenko of Northeastern University (USA) and Professor Alexander Shashkin of the Institute of Solid State Physics (Russia) study two-dimensional electron systems. In this field, the behaviour of electrons under different environmental conditions alters the macroscopic properties of the materials they constitute. For example, some metallic compounds transition from an insulating state to a metallic, conductive state as the system parameters change. Understanding precisely how and why this occurs may be crucial to developing the next generation of nanoscale materials, such as room-temperature superconductors.
Understanding the Interactions of Electrons
Professor Sergey Kravchenko (Northeastern University, USA) and Professor Alexander Shashkin (Institute of Solid State Physics, Russia) have fruitfully collaborated for several decades. Together, they are credited with discovering metal-insulator transitions in two-dimensional electron systems – listed by the American Physical Society’s ‘A Century of Mesoscopic Physics (1899–1999)’ as one of the 50 most important discoveries of the last century in this field.
Their research is all about electrons – the negatively charged particles that orbit the positively charged nuclei of atoms. Outside of nuclear reactions like fusion and fission, the behaviour of electrons determines all chemical reactions.
To anthropomorphise for a moment, matter and energy ‘like’ to be comfortable. That is, they like to find low-energy states. Take, for example, a marble at the top of a hill – it has a lot of gravitational potential energy, which is transformed into kinetic energy as it rolls down the hill, till it rests in the valley, a lower energy state. When two atoms form molecules (such as when one oxygen and two hydrogen atoms form H2O), it’s generally because the resulting molecule is more stable. Less energy is bound up in the system, and so this energy is released.
There are three primary types of chemical bond: covalent, metallic and ionic. In ionic bonding, atoms with opposite charges attract and bind to each other. In covalent bonding, multiple atoms ‘share’ an electron or two, keeping them together.
While organic chemistry is focused on covalent bonding between elements like hydrogen, carbon, oxygen and nitrogen (collectively constituting 96% of your body mass), as well as some ionic bonding in molecules like salt, most elements on the periodic table are metals and bond through metallic bonds. It is this type of structure that Professor Kravchenko and Professor Shashkin are particularly interested in.
In metallic bonding, atoms form a regular, repeating structure where outer electrons are shared collectively. In contrast to covalent bonding, the electrons in metallic bonding can become delocalised, meaning they aren’t bound to particular atoms. This is why metals can be very effective conductors of heat and electricity, as the delocalised electrons can carry current and heat energy.
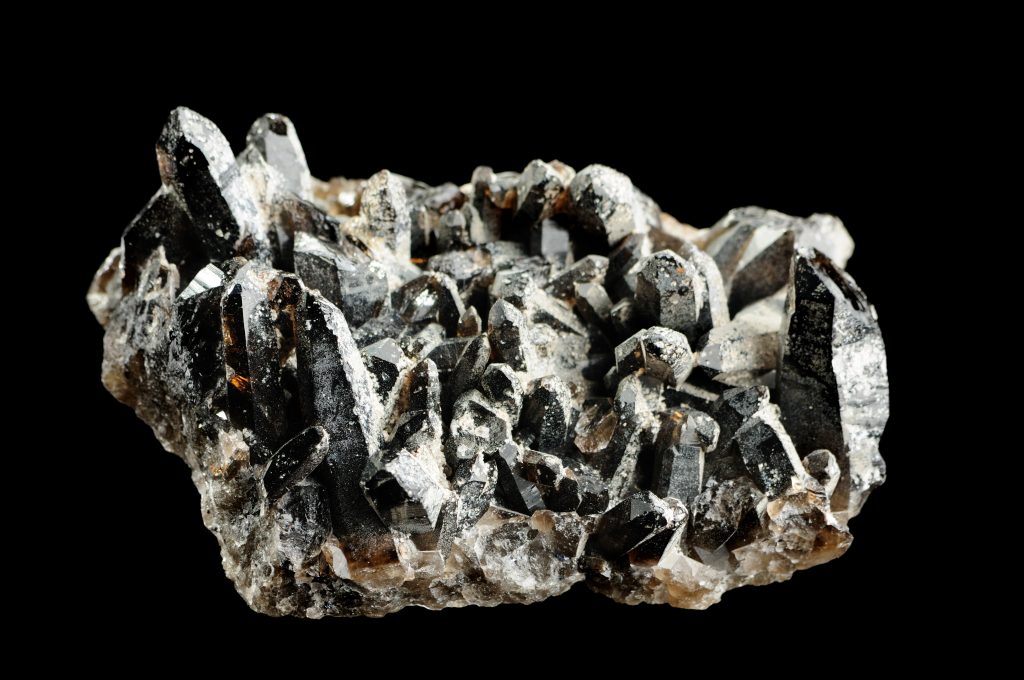
Magnetite.
The Transition Between Metal and Insulator
While most metals at room temperature are great conductors, this isn’t universal. Professor Kravchenko and Profesor Shashkin study certain systems where environmental conditions, such as temperature or the presence of strong magnetic fields, alter the macroscopic properties of the material by changing how the electrons behave.
This field is fascinating because we don’t fully understand the conditions under which such transitions occur. Professor Kravchenko and Professor Shashkin are dedicated to developing much-needed insight into this kind of transition.
Their focus is on strongly interacting two-dimensional electron systems. These are structures where electrons are confined to move in only two dimensions, and where the interactions between electrons dominate the behaviour of the material.
Generally, the more ordered the structure of a molecule, the more tightly constrained the electrons are. If the structure becomes more disordered, the electrons are less constrained and become more delocalised, increasing their capacity to conduct electricity and heat.
For example, magnetite is a naturally occurring magnetic iron ore. This is where we get the word ‘magnet’, as these naturally magnetic stones were called stones of Magnesia, named after the Greek region where magnetite was mined. Below around -153 degrees Celsius, magnetite is an insulator, as the iron ions are arranged in a way that localises the electrons to some degree. Heated above this temperature, the crystal structure changes from a repeating parallelogram prism to a repeating cubic structure, and the regularity in the iron ion arrangement breaks down. This means the electrons become disordered and delocalised, and the material becomes metallic-like and conductive.
Many materials undergo transitions between metallic behaviour and insulating behaviour, collectively called metal-insulator transitions. These are especially important in nanotechnology and computing, as field-effect transistors – one of the basic building blocks of modern electronics – transition between a conductive and insulating state when subjected to an electric field perpendicular to the two-dimensional electron system.
As Professor Kravchenko and Professor Shashkin point out, these types of transition are great test beds for studying how electrons behave in the presence of disorder at temperatures near absolute zero. Prior to their experiments, a transition from an insulator to a metal was considered impossible in two-dimensional electron systems, following the seminal 1979 theoretical paper by Abrahams, Anderson, Licciardello and Ramakrishnan. However, the theorists considered non- or weakly-interacting electrons. In the experiments of Professors Kravchenko and Shashkin, the energy of electron-electron interactions by far exceeds their kinetic energy and obviously cannot be ignored, and so, the physics becomes entirely different.
Band Flattening
Electrons are organised into energy bands, levels that the electrons can occupy. Think of it like a ladder, where each rung represents a different energy level that an electron can have. In some materials, like metals, the upper energy band is not completely filled, with the maximum energy called the ‘Fermi energy’ (Fermi level). The electrons that move with the energy equal to the Fermi energy may, in principle, have a larger effective mass compared to the ‘average’ electron mass. This is related to the so-called ‘band flattening’ whereby the energy spectrum becomes flat, i.e., independent of the momentum.
Band flattening is predicted to occur in several systems, but the researchers focused on two-dimensional electron systems. This is when the electrons are confined to only a two-dimensional plane or sheet, only a few atoms thick.
When electrons are confined in a small space, their behaviour changes, and this affects the material’s macroscopic properties. The researchers used a structure known as a quantum well to achieve this confinement. The walls of the well are made of a different material, which can trap the electrons in between.
Professor Kravchenko, Professor Shashkin and their collaborators studied band flattening by building ultra-high quality quantum wells from silicon sandwiched between layers of silicon germanium. The silicon in the middle has a lower potential energy, and so this acts as the well, confining electrons.
In such materials, the properties of the electrons can change in unexpected ways, for example, by behaving as if they had a lower mass. Further, when studying these changes, experiments have found cases where the effect contradicts intuitive expectations and calculations.
By probing just how these materials and their properties change under different conditions, such as differing temperatures or electron densities, they hope to improve our understanding of electron behaviour and reconcile the contradicting theoretical predictions and experimental data.
The results are hard to explain intuitively, but they found that with decreasing electron density (corresponding to increasing strength of interactions), the effective mass of the electrons steadily increases, while at low electron densities, the average mass of the electrons stops increasing and approaches a constant value, while the mass of the electron on the Fermi level continues to grow. Crucially, they argue that the results can only be attributed to the different behaviours of the electrons that have the ‘average’ mass and those at the Fermi level, indicating that band flattening has indeed occurred.
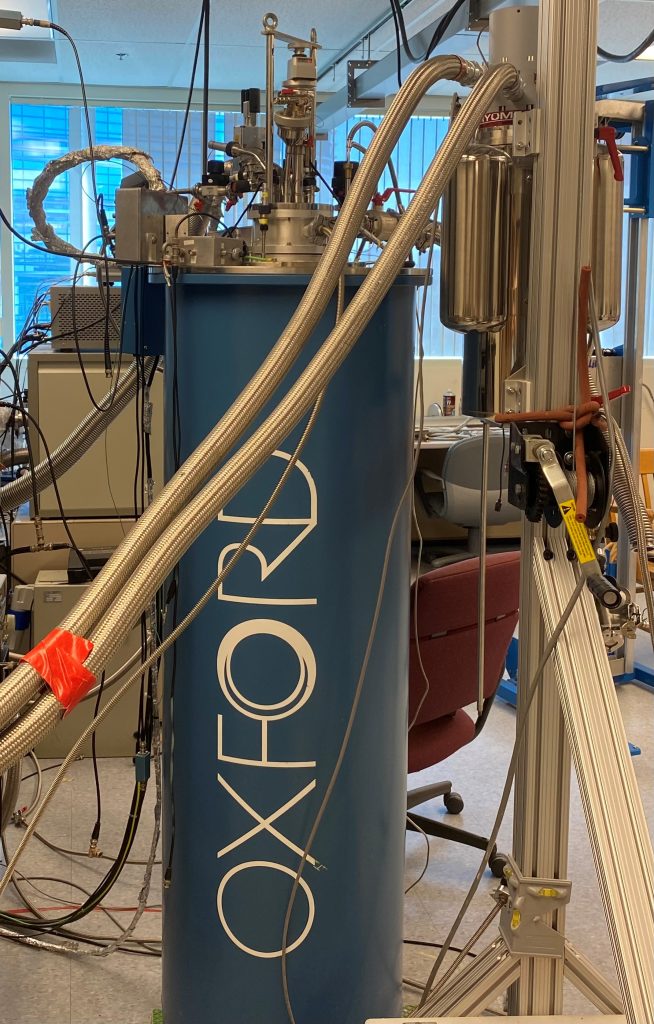
Oxford dilution refrigerator capable of producing temperatures down to 18 millikelvins. Credit Sergey Kravchenko
Searching for Illusive Quantum Crystals
In a third paper, Professor Kravchenko and Professor Shashkin report a breakthrough in understanding the origin of the low-density state in a strongly interacting two-dimensional electron system in a certain kind of semiconductor transistor.
They point out that while the metallic side of metal-insulator transitions has been studied previously, the insulating side isn’t well understood. At extremely low electron densities, near what’s called the strongly-interacting limit, theories predict that the electrons crystallise into a quantum Wigner crystal. This is a strange and exotic state of matter whereby properties are determined by quantum-mechanical effects. This occurs because the potential energy of the electrons dominates over their kinetic energy at low densities, and so the specifics of their spatial arrangement become increasingly important. To achieve their ‘desired’ minimal energy state, they form a regular lattice, a repeating triangular structure.
Despite this attractive theoretical prediction, researchers haven’t found any definitive evidence for the formation of quantum Wigner crystals in this situation. Professor Kravchenko and Professor Shashkin studied this phenomenon in a type of common transistor called a MOSFET (metal–oxide–semiconductor field-effect transistor), and observed two-threshold voltage-current characteristics, which displayed a dramatic increase in noise (random variations) between the two threshold voltages. This double-threshold behaviour is exotic and can’t be explained with traditional models but is strikingly similar to that observed previously in the special case of collective depinning of the vortex lattice in a certain type of superconductor. By adapting the models used for such vortexes to the two-dimensional electron solids, the researchers were able to match the theory to their experimental results, supporting the idea that the quantum electron solid is the origin of the low-density state.
Professor Kravchenko and Professor Shashkin have worked at the cutting edge of solid state physics for decades, delving deep into the world of two-dimensional electron systems. They’ve discovered several new and exciting behaviours that shed light on the bizarre and unintuitive state transitions that materials undergo in extreme environments. Their ultra-precise measurements have allowed them to identify new behaviours and new constraints on the theoretical underpinnings of electron behaviour. Their research has furthered our understanding of a field that may be the key to the next generation of high-tech materials, such as even small transistors and even room-temperature superconductors.
SHARE
DOWNLOAD E-BOOK
REFERENCE
https://doi.org/10.33548/SCIENTIA943
MEET THE RESEARCHERS
Professor Sergey Kravchenko
Physics Department
Northeastern University
Boston, MA
USA
Professor Sergey Kravchenko is a professor at Northeastern University in Boston, Massachusetts. In 1982, he obtained his MSc at the Moscow Institute of Physics and Technology in Russia, and then focused his attention on solid state physics, obtaining his PhD in 1988 from the Institute of Solid State Physics in Chernogolovka, Russia. He has maintained a highly active research profile working at several institutes in Russia, the UK and the USA. Professor Kravchenko’s contributions to science have led to the award of several distinguished honours, including being elected as a Fellow of the American Physical Society in 2008. His research focuses on two-dimensional electron systems, a field in which he has made several notable discoveries.
CONTACT:
E: s.kravchenko@northeastern.edu
W: https://cos.northeastern.edu/people/sergey-kravchenko/
Professor Alexander Shashkin
Institute of Solid State Physics
Russian Academy of Sciences
Chernogolovka
Russia
Professor Alexander Shashkin is the Head of Laboratory at the Institute of Solid State Physics, part of the Russian Academy of Sciences in Chernogolovka. In 1984, he obtained his MSc in General and Applied Physics from the Moscow Institute of Physics and Technology, and then specialised in two-dimensional electron systems during his PhD completed at the Institute of Solid State Physics in 1989. He went on to receive a DSc from the same institute in 2008, where he focused on metal-insulator transitions in two-dimensional electron systems. Professor Shashkin has conducted an impressive body of research and co-authored more than 100 published papers in solid state physics.
CONTACT
W: http://www.issp.ac.ru/lqt/index_en.php
KEY COLLABORATORS
VT Dolgopolov (Institute of Solid State Physics, Russia)
TM Klapwijk (Delft University of Technology, The Netherlands)
CW Liu (National Taiwan University)
M Yu Melnikov (Institute of Solid State Physics, Russia)
MP Sarachik (City College of the City University of New York, USA)
FUNDING
Professor Kravchenko is funded by NSF Grant No. 1904024, and Professor Shashkin is funded by RSF Grant No. 22-22-00333.
FURTHER READING
MY Melnikov, AA Shashkin, VT Dolgopolov, et al., Quantum phase transition in ultrahigh mobility SiGe/Si/SiGe two-dimensional electron system, Physical Review B, 2019, 99, 081106(R). DOI: https://doi.org/10.48550/arXiv.1808.10063
P Brussarski, S Li, SV Kravchenko, et al., Transport evidence for a sliding two-dimensional quantum electron solid, Nature Communications, 2018, 9(1), 3803. DOI: https://doi.org/10.1038/s41467-018-06332-9
MY Melnikov, AA Shashkin, VT Dolgopolov, et al., Indication of band flattening at the Fermi level in a strongly correlated electron system, Scientific Reports, 2017, 7, 14539. DOI: https://doi.org/10.1038/s41598-017-15117-x
REPUBLISH OUR ARTICLES
We encourage all formats of sharing and republishing of our articles. Whether you want to host on your website, publication or blog, we welcome this. Find out more
Creative Commons Licence (CC BY 4.0)
This work is licensed under a Creative Commons Attribution 4.0 International License.
What does this mean?
Share: You can copy and redistribute the material in any medium or format
Adapt: You can change, and build upon the material for any purpose, even commercially.
Credit: You must give appropriate credit, provide a link to the license, and indicate if changes were made.
SUBSCRIBE NOW
Follow Us
MORE ARTICLES YOU MAY LIKE
Professor Giorgio Buttazzo | Artificial Intelligence and a Crossroads for Humanity
Where do we stand with artificial intelligence? Might machines take over our jobs? Can machines become conscious? Might we be harmed by robots? What is the future of humanity? Professor Giorgio Buttazzo of Scuola Superiore Sant’Anna is an expert in artificial intelligence and neural networks. In a recent publication, he provides considered insights into some of the most pressing questions surrounding artificial intelligence and humanity.
Dr Olalla Castro-Alvaredo | Measuring Entanglement: Symmetry-Resolved Entropy
Dr Olalla Castro-Alvaredo of the City University of London (UK) and her collaborators are advancing our understanding of an important phenomenon of quantum mechanical systems known as entanglement and, especially, its mathematical measures. Symmetry-resolved entanglement entropy is one such measure. Their study focuses on special quantum states which are excited with respect to a ground state. The research shows how the entanglement amongst quantum particles can be measured and assesses the contribution to the entanglement of quasiparticle excitations, particularly in the presence of additional symmetries.
Professor Martin Trefzer | Bridging Nature and Artificial Intelligence for Smart Electronics Technology
The ever-developing world of artificial intelligence (AI) stands at the tip of a transformative breakthrough. Professor Martin Trefzer from the University of York and Professor Jim Harkin from Ulster University have introduced a revolutionary approach to neural network design. They work on an electronic system based on AI that forms the basis of the cross-disciplinary project called Nervous Systems, which aims to build electronic neuromorphic devices with an artificial intelligence system mirroring the adaptability and responsiveness of biological neural systems.
Dr Jon Tore Lieng | Dynamically Installed Anchors for Floating Offshore Turbines
Effectively harnessing offshore wind presents a valuable opportunity to increase energy supplies. Floating wind turbines present several advantages over traditional fixed turbines in more shallow waters. Dr Jon Tore Lieng from Deep Sea Anchors and colleagues have developed a type of dynamically installed anchor to hold the structures in place while reducing both the costs and complexity associated with installation where cohesive seabed sediments are realised.